Vol. 74, No. 9 (2025)
2025-05-05
VIEWS AND PERSPECTIVES
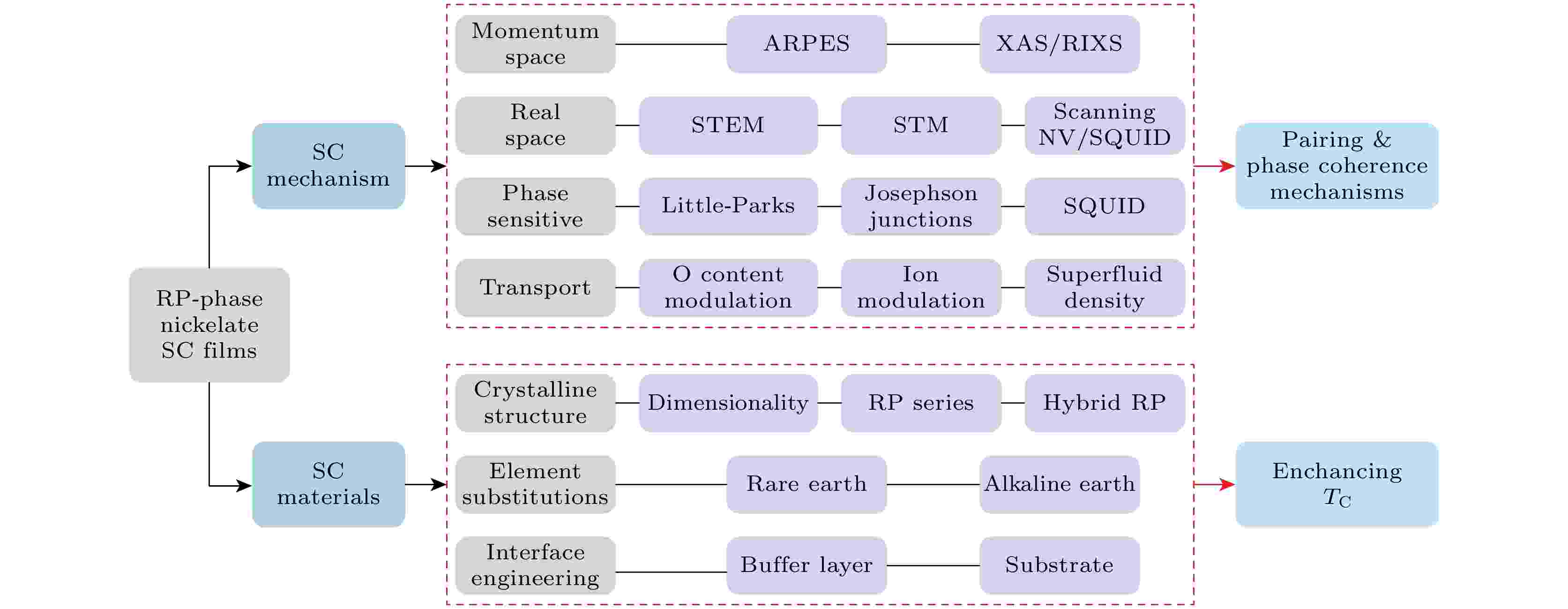
EDITOR'S SUGGESTION
2025, 74 (9): 097401.
doi: 10.7498/aps.74.20250331
Abstract +
In recent years, significant progress has been made in the superconductivity of nickelates, with global teams discovering various nickelate superconductors under ambient and high pressure conditions. Research teams in China and USA have independently discovered ambient-pressure superconductivity in Ruddlesden-Popper bilayer nickelate thin films through different technical pathways, establishing a novel platform for probing high-temperature superconducting mechanisms. The Chinese teams have synthesized pure-phase bilayer nickelate films with atomically smooth surfaces by using their proprietary Gigantic-Oxidative Atomic-Layer-by-Layer Epitaxy (GOALL-Epitaxy) technique. After in situ strong oxidation processing of surface, surface-sensitive measurements, such as ARPES, can be conducted on these atomically flat films to reveal the electronic structure of the superconducting phase, and further in-depth experimental research on superconducting mechanisms is expected. Through synergistic efforts in lattice engineering, rare-earth/alkaline-earth element substitution, and interface strain engineering, this system has the potential to achieve higher superconducting transition temperatures.
SPECIAL TOPIC—Dynamics of atoms and molecules at extremes
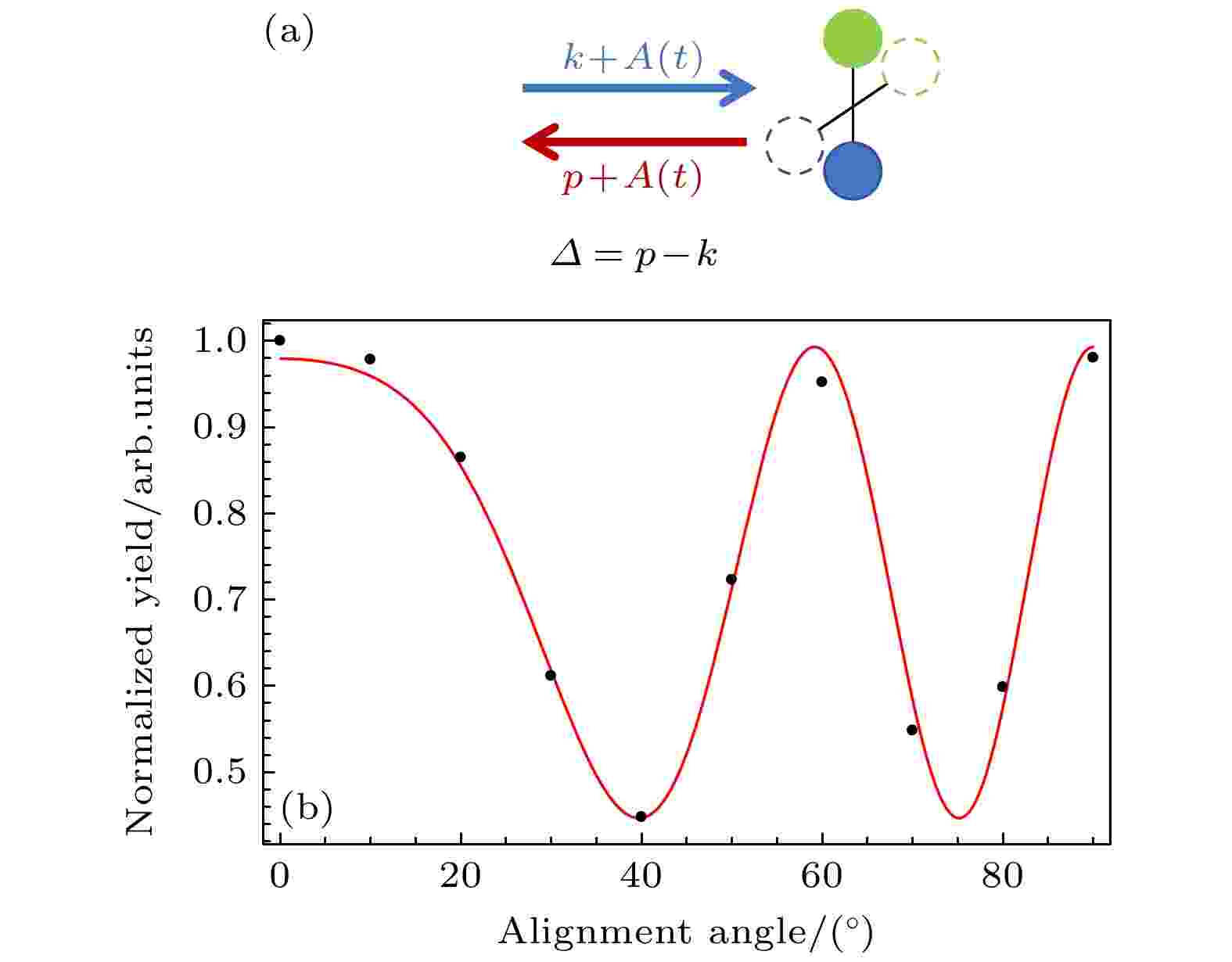
EDITOR'S SUGGESTION
2025, 74 (9): 093201.
doi: 10.7498/aps.74.20241792
Abstract +
The study of atomic and molecular structure imaging is of great significance in revealing the microscopic nature of matter and promoting the frontier development of materials science and life science. The rapid development of femtosecond laser technology provides a new method for detecting atomic and molecular structures on an ultrafast time scale, such as strong-field tomography scheme. Strong-field tomography uses strong-field driven electron rescattering to detect the structure of oriented molecules. The advantage of this scheme is that it does not require priori knowledge of atomic differential cross-sections. Using the strong-field tomography scheme, the structural extraction of homonuclear diatomic molecules can be realized successfully. However, it is currently unclear whether this imaging scheme is applicable to the heteronuclear molecular system with more complex cross section of the electron. In this work, heteronuclear diatomic molecules are taken for example and the strong-field tomography scheme is used to study the imaging of the molecular structure. By solving the time-dependent Schrödinger equation, the variation of the photoelectron yield with the orientation angle of the molecular axis is obtained. Next, a fitting method for the variation of the photoelectron yields of the heteronuclear molecules with the orientation angle is presented, and then the fitted value of the internuclear separation is obtained. It is found that the fitting result is comparable to the real molecular internuclear separation, indicating that the strong-field tomography scheme is also suitable for the extraction of heteronuclear molecular structural information.
SPECIAL TOPIC—Ultrafast physics in atomic, molecular and optical systems
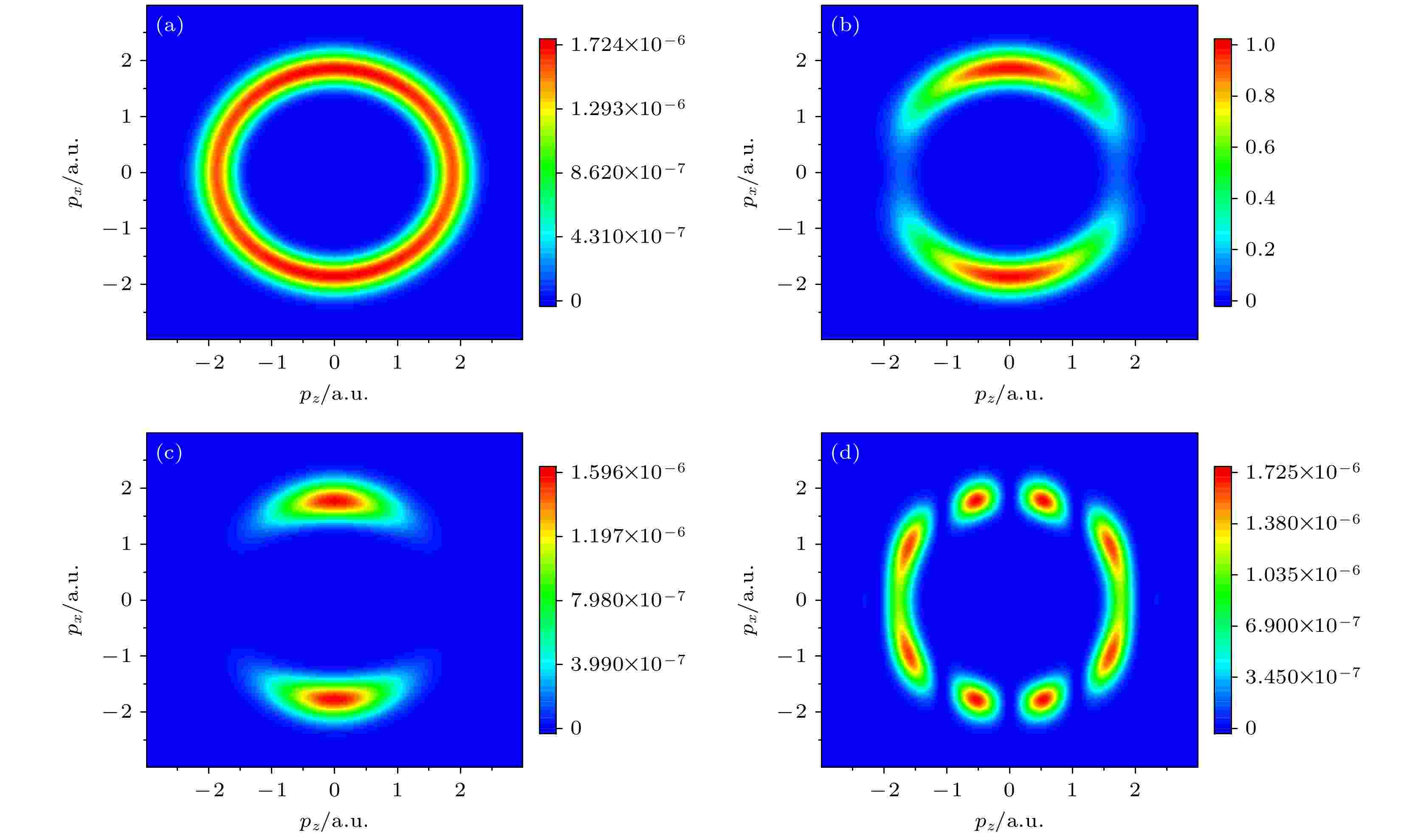
EDITOR'S SUGGESTION
2025, 74 (9): 093202.
doi: 10.7498/aps.74.20250064
Abstract +
Despite the molecular strong-field approximation (SFA) theory has made remarkable achievements in describing the ultrafast dynamics of molecules in intense laser fields, there are basic inconsistencies in the theory itself. On the one hand, the basic principle of SFA requires that the initial state be an eigenstate of the system in the absence of the field, and on the other hand, the spatial translation invariance of the physical process requires that the initial state of the system be a laser-field-dressed state. These two conflicting requirements correspond to the two forms of molecular SFA theories, namely, the undressed state and the dressed state. The two theoretical validity and applicability conditions are widely disputed. In this paper, we investigate the ionization processes of N2 and Ne2 molecules in an elliptically polarized laser field and a circularly polarized laser field, aiming to solve the above-mentioned controversies. Elliptically polarized laser can efficiently suppress the re-scattering process and the influence of various interference effects, which makes the ionization process cleaner, and thus can effectively screen the applicable conditions for the dressed and undressed states. We calculate the photoelectron momentum distributions corresponding to different molecular orbitals in the dressed and undressed states by using the SFA and the Coulomb-corrected strong-field approximation and compare them with previous experimental results. For molecules with large nuclear spacing such as Ne2, we find that the dressed state is necessary to accurately characterise their ionization, however, for molecules with small nuclear spacing such as N2, the dressed state description is inapplicable. The conclusions of this work provide a reference for accurately describing laser-induced molecular ultrafast processes and further developing corresponding theories and molecular ultrafast imaging schemes.
SPECIAL TOPIC—Instrumentation and metrology for ultrafast atomic and molecular spectroscopy
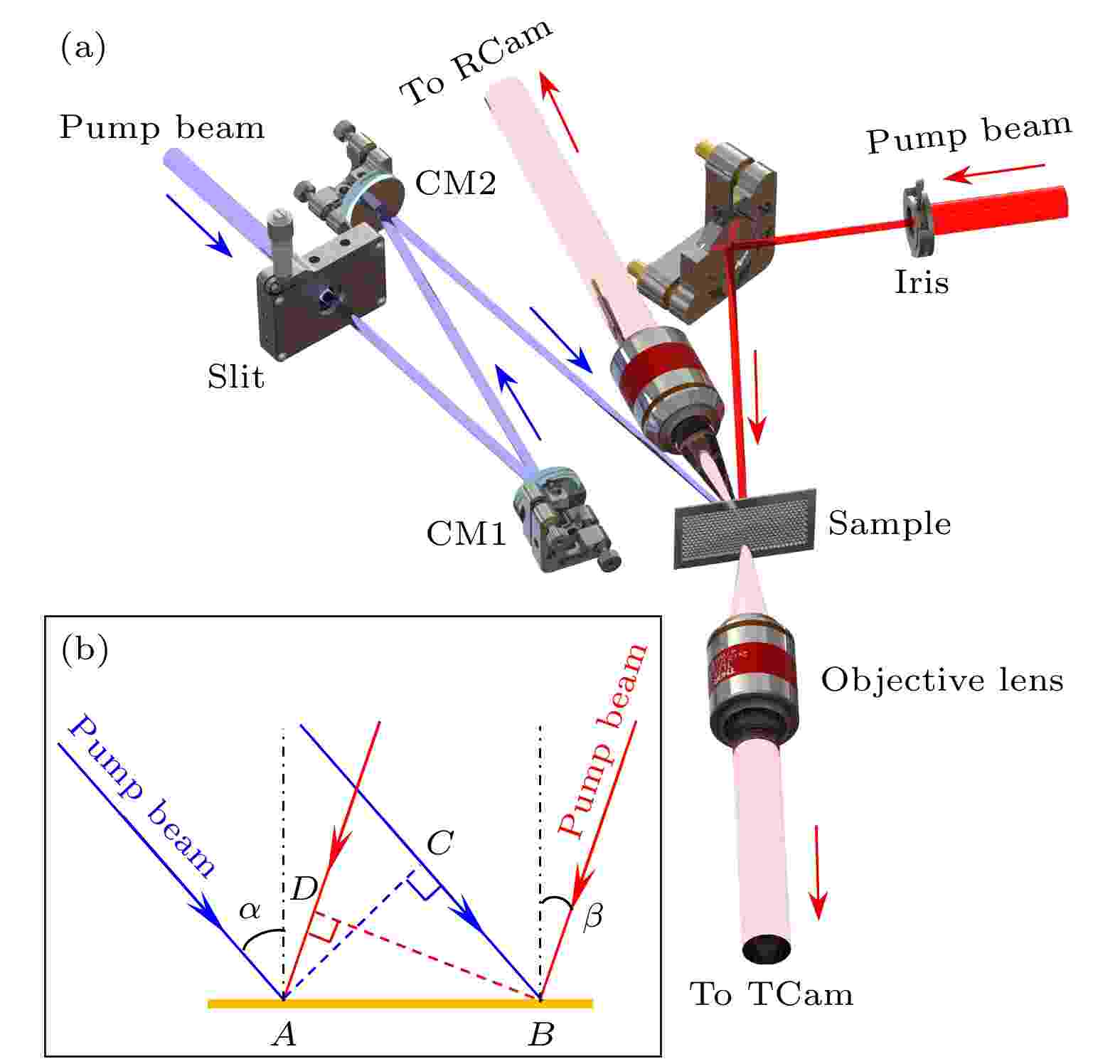
EDITOR'S SUGGESTION
2025, 74 (9): 095202.
doi: 10.7498/aps.74.20250135
Abstract +
The spatial chirp based single-shot pump-probe technique represents a pivotal technology for studying electron non-equilibrium dynamics in warm dense matter created with intense laser pulses. Notably, its time resolution can reach tens of femtoseconds. In this work, we introduce the single-shot measurement technique of ac conductivity of warm dense matter, as well as a detailed account of the experimental setup. In addition, the main factors limiting the time resolution of the system are discussed in depth. We show the system can achieve a resolution of 13.8 femtoseconds. Nevertheless, during practical application, several aspects, namely the calibration of the zero-delay, the depth of field of the imaging system, and the low-pass filtering effect inherent in the imaging system, will exert a substantial influence on the time-resolution. This research has important reference for enhancing the time accuracy of single-shot measurement of ac conductivity of warm dense matter. Moreover, it serves as a potent tool for the in-depth study of the ultrafast dynamic processes of materials under strong-field conditions.
REVIEW
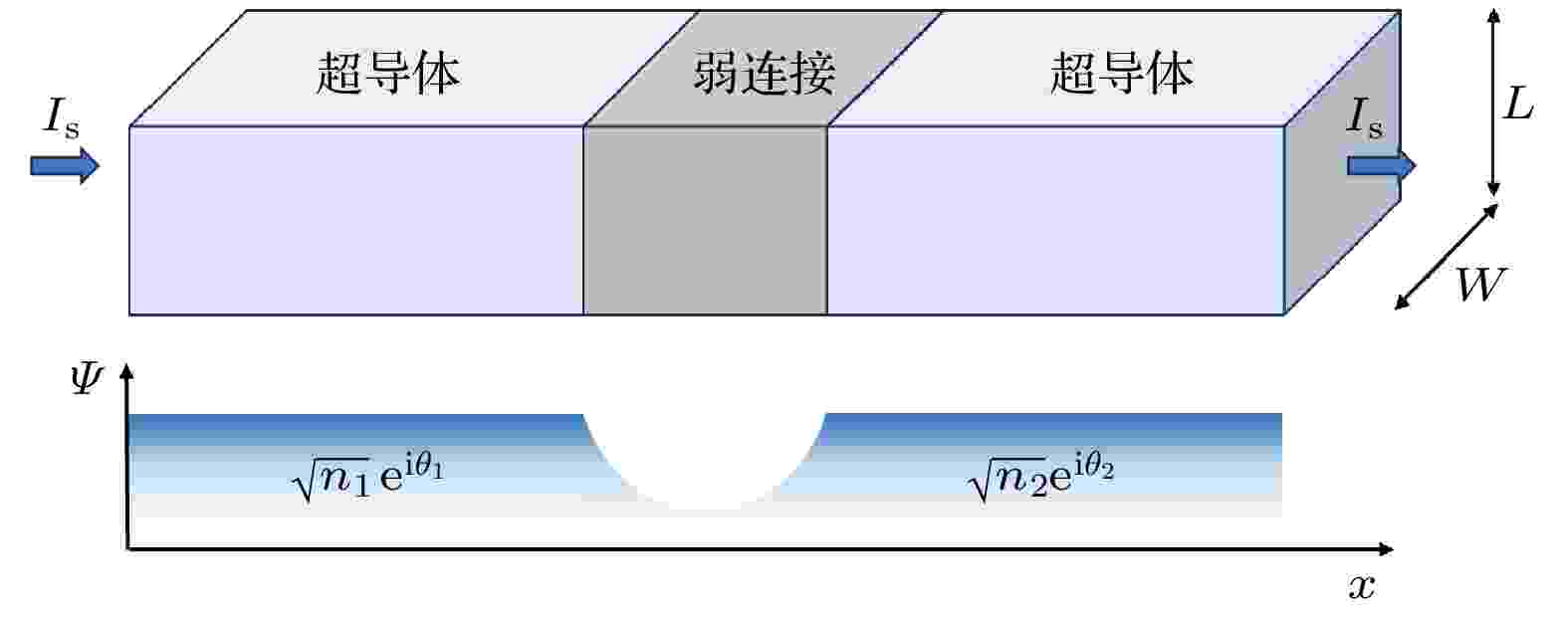
EDITOR'S SUGGESTION
2025, 74 (9): 090304.
doi: 10.7498/aps.74.20241262
Abstract +
This paper reviews the physical principles, development history of related application research, current research status and prospects of the Josephson voltage standard (JVS) working at liquid helium temperatures. The JVS working at liquid helium temperature has advantages of high mobility and low-energy consumption, and has a broad application prospect. This paper describes the research status of Josephson voltage standards, focusing on the possibility of developing a JVS based on high-temperature superconductors, and the challenges in chip preparation. In addition, a newly developed preparation technology for Josephson junction, namely the focused helium ion beam, is introduced. It has advantages in the preparation of high consistent Josephson junction arrays in high consistency. Therefore, it is a possible technical route for exploring the realization of JVS working at liquid helium temperature in the future.
GENERAL
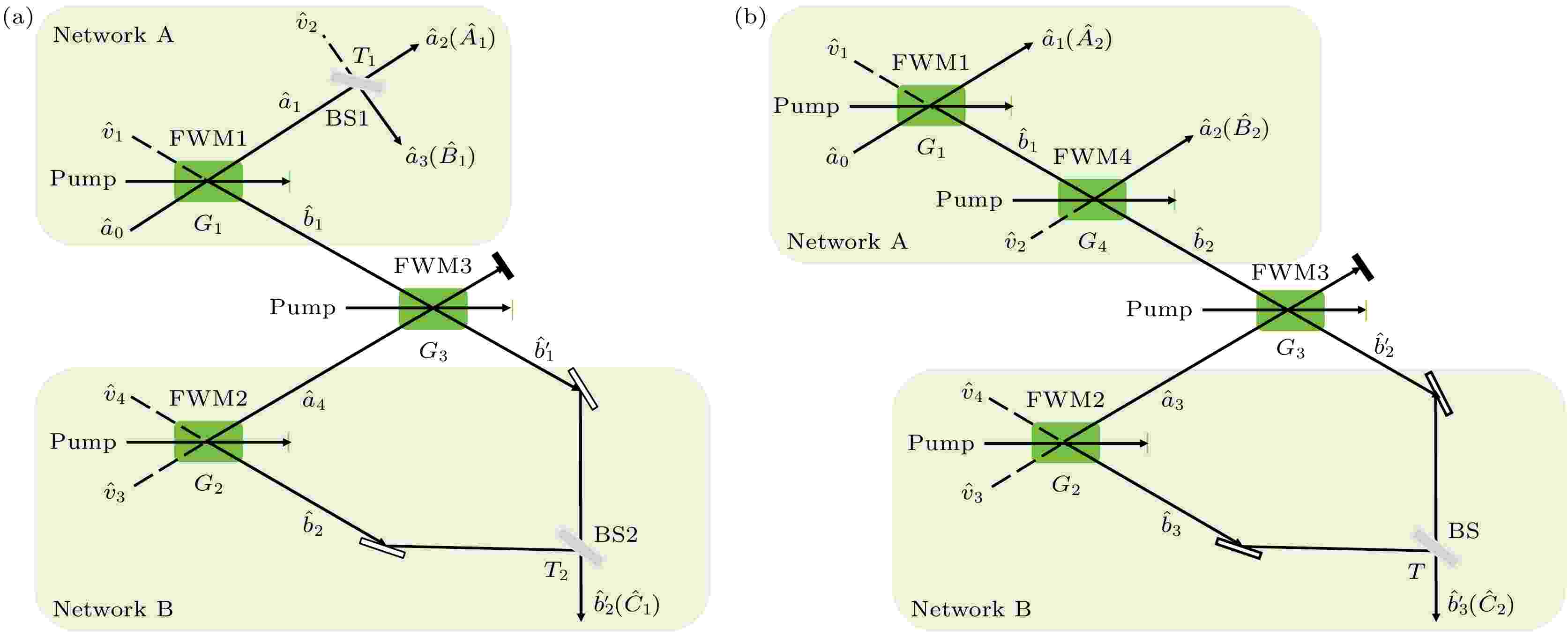
EDITOR'S SUGGESTION
2025, 74 (9): 090301.
doi: 10.7498/aps.74.20250083
Abstract +
Quantum resource swapping is crucial for establishing quantum networks and achieving efficient quantum communication and it allows quantum resources to be shared and allocated between nodes in a quantum network, thereby enhancing network flexibility and quantum information processing capabilities. Quantum steering is a special type of quantum correlation that exhibits unique asymmetry compared with quantum entanglement and Bell nonlocality. This asymmetry enables quantum steering swapping to establish one-way or two-way asymmetry quantum steering between two independent optical modes, which is crucial for constructing asymmetric quantum networks. In this work, an all-optical quantum steering swapping scheme is proposed based on tripartite entangled state and bipartite entangled state. The all-optical scheme does not involve optic-electro conversion nor electro-optic conversion, but utilizes a low-noise, high-bandwidth four-wave mixing process to achieve the function of Bell state measurement in traditional schemes without measurement. After the steering swapping operation, the two originally independent entangled states without direct interaction generate quantum steering. In this work, two swapping schemes in the four-wave mixing processes, combined with linear beam splitter and nonlinear beam splitter, are investigated. By analyzing the steering characteristics of the output modes, both schemes exhibit varieties of multipartite steering types. By adjusting the transmissivity of the linear beam splitter and the gain of the four-wave mixing process, the steering relationship can be flexibly manipulated to achieve one-way and two-way asymmetry steering. This provides new possibilities for one-way quantum communication and quantum information processing, making the utilization of quantum resources more efficient and controllable. Through in-depth analysis of the steering characteristics after swapping, it is found that compared with the linear beam splitter scheme, the nonlinear beam splitter scheme not only significantly improves the capability of quantum steering, but also allows for more flexible manipulation of monogamy relations of quantum steering. By optimizing the gain parameters of the nonlinear beam splitter, the precise manipulation of the monogamy relations can be achieved over a wider range. This not only expands broader application prospects for information processing and quantum communication in quantum networks, but also lays an important foundation for building efficient and secure quantum information processing systems.
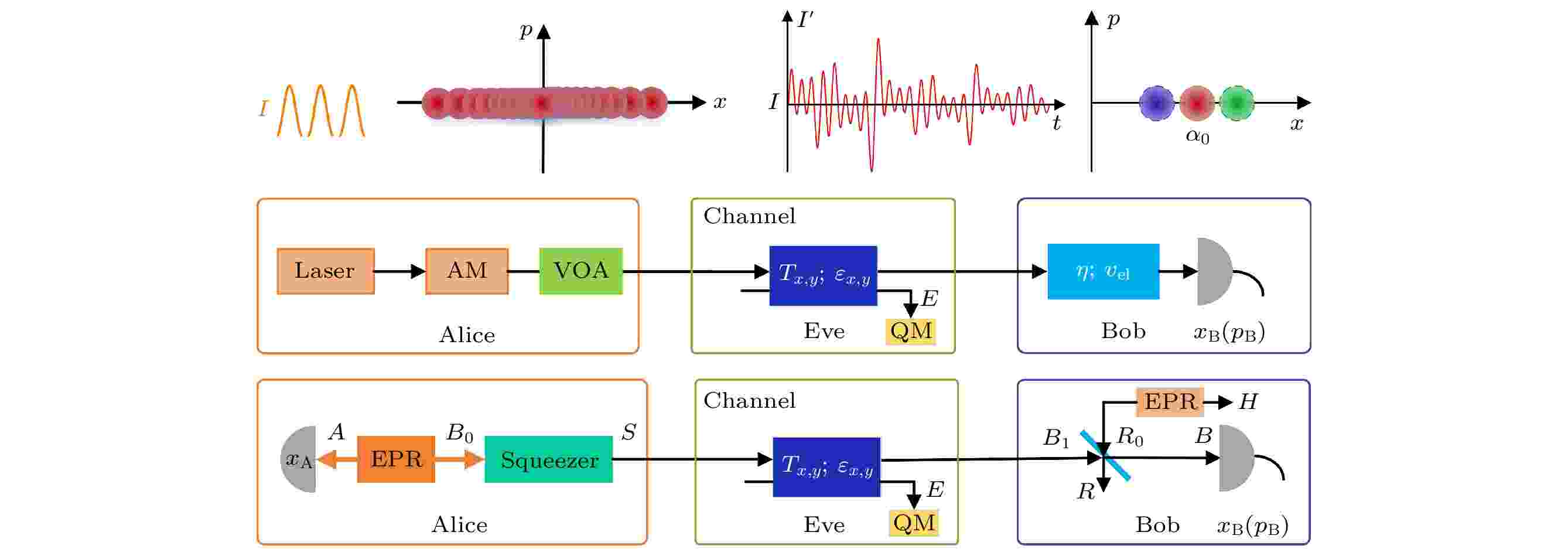
2025, 74 (9): 090302.
doi: 10.7498/aps.74.20250025
Abstract +
Unidimensional Gaussian modulation continuous-variable quantum key distribution (UD CV-QKD) uses only one modulator to encode information. The UD CV-QKD has the advantages of low implementation cost and low random number consumption, making it attractive for the construction of future miniaturized and low-cost large-scale quantum communication networks. However, in the actual application of the protocol, the intensity fluctuation of the source pulsed light, device defects, and external environmental interference maybe lead to the generation of source intensity errors, thereby affecting the realistic security and performance of the protocol. To solve these problems, the security and performance of UD CV-QKD are studied in depth under source intensity errors in this work. The mechanism of source intensity errors influencing the protocol parameter estimation process is analyzed. To make it possible that the protocol can operate stably under various realistic conditions and ensure communication security, three practical assumptions about the sender’s abilities are made in this work, and corresponding data optimization processing schemes for these assumptions are proposed to reduce the negative influence of source intensity errors. Additionally, both source errors and finite-size effect are comprehensively considered to ensure the realistic security of the system. The simulation results indicate that the source intensity errors cannot be neglected and the maximum transmission distance of the system will be reduced by approximately 20 km for significant intensity fluctuations. Therefore, in the practical implementation of the protocol, the influence of source intensity errors must be fully considered, and the corresponding countermeasures should be taken to reduce or even eliminate these errors. This study provides theoretical guidance for securely implementing the UD CV-QKD in real-world environments.
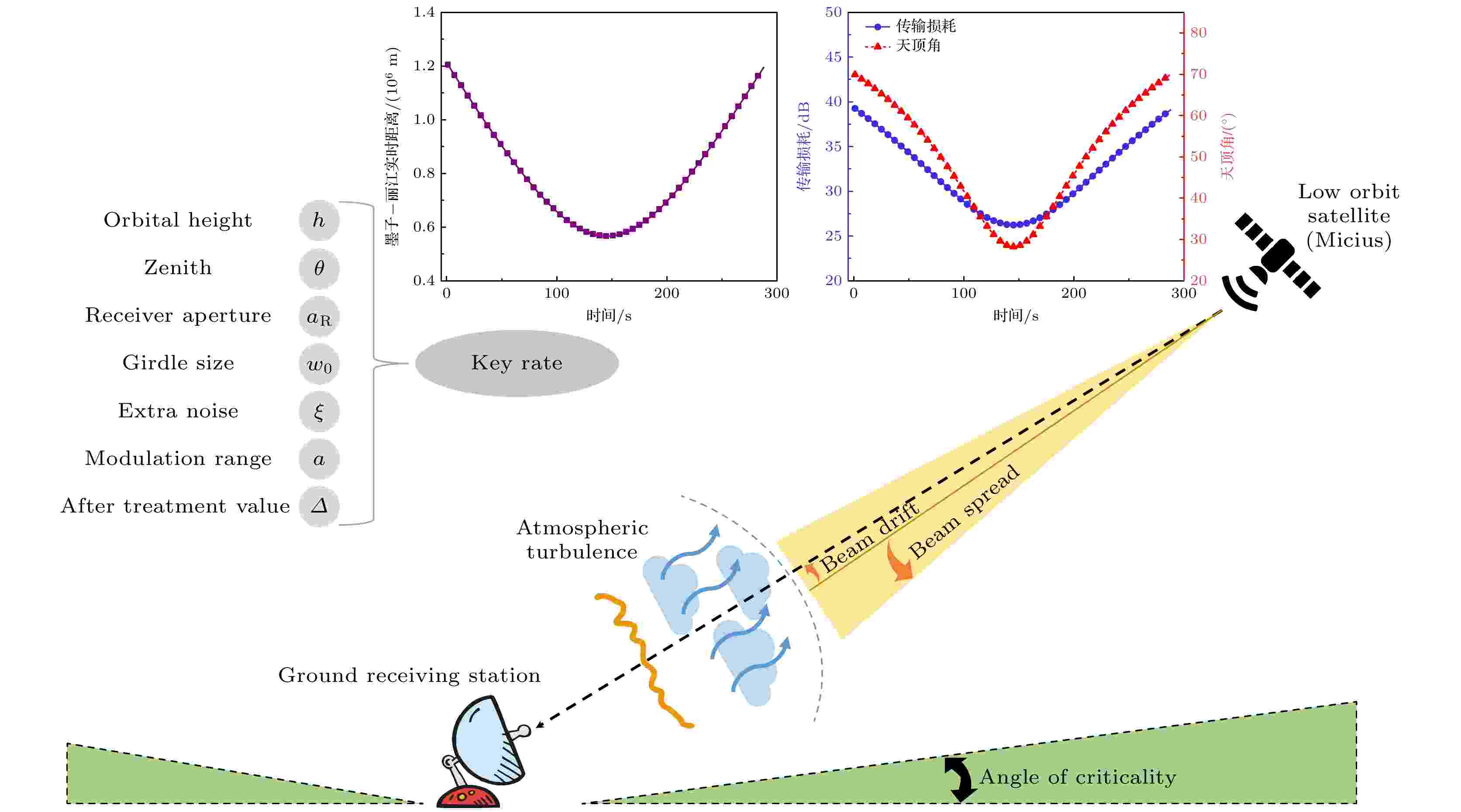
EDITOR'S SUGGESTION
2025, 74 (9): 090303.
doi: 10.7498/aps.74.20241682
Abstract +
Continuous variable quantum key distribution (CV-QKD) has emerged as a promising candidate for quantum-secure communication due to its experimentally demonstrated high key rates in fiber-optic channels. However, the feasibility of discrete modulation CV-QKD in satellite-to-ground downlinks remains an open question due to practical challenges such as high transmission loss, limited communication windows, and atmospheric turbulence. In this work, a comprehensive framework is proposed to evaluate the feasibility of discrete modulation CV-QKD by integrating orbital dynamics and atmospheric channel models, and to comprehensively analyze the influence of the parameter space on free-space discrete modulation CV-QKD. To achieve this, a free-space CV-QKD simulation platform is employed, which calculates the elevation angle and transmission distance based on precise orbital models, thereby providing a more practical assessment of the key rate for discrete modulation CV-QKD. Simulation results verify the feasibility and practicality of discrete modulation CV-QKD in satellite-based quantum communication systems. Furthermore, the critical factors influencing the key rate performance are identified, and parameter optimization strategies are proposed, providing theoretical support for realizing the future satellite-to-ground discrete modulation CV-QKD.
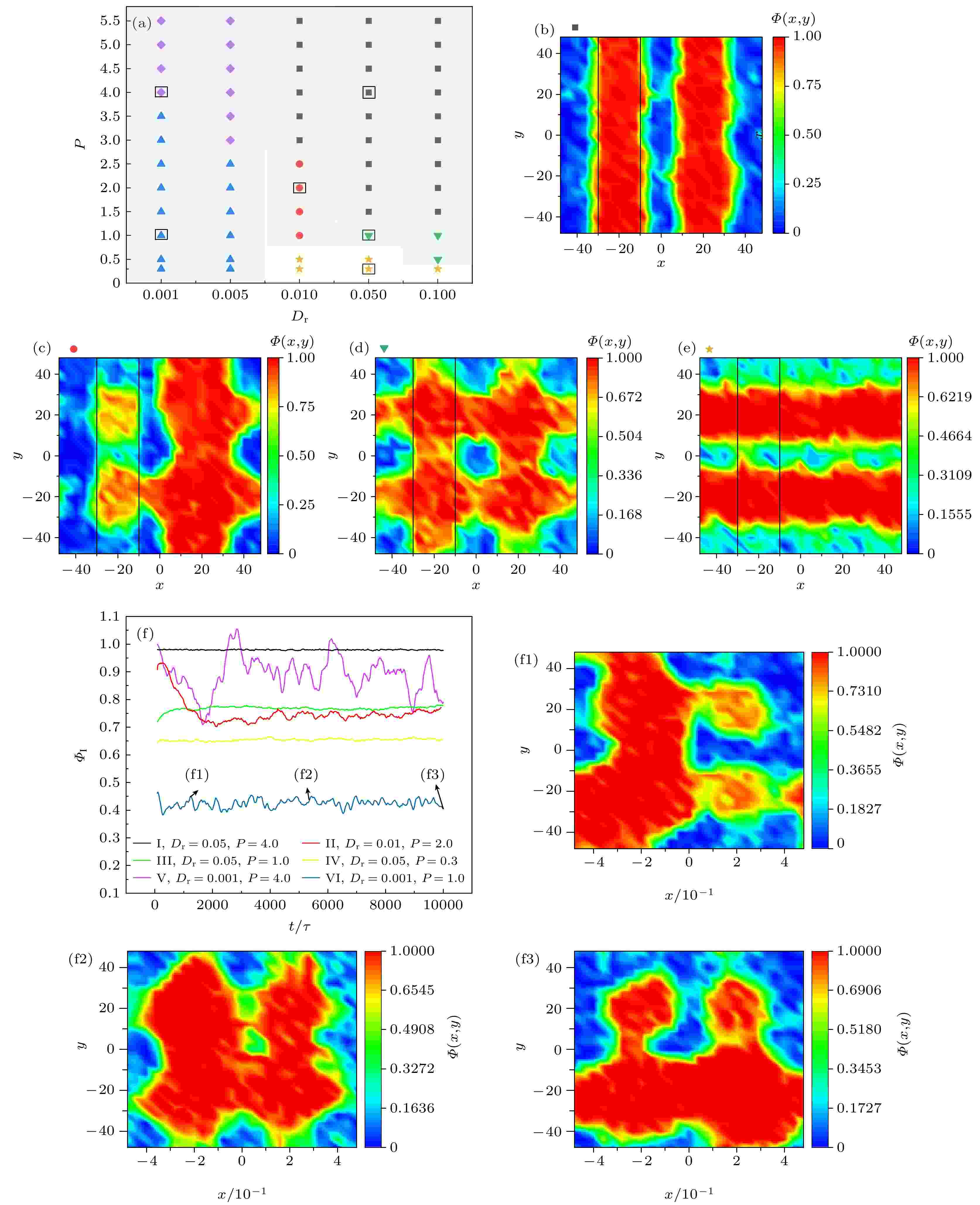
2025, 74 (9): 090501.
doi: 10.7498/aps.74.20241556
Abstract +
Active particle systems are nonequilibrium systems composed of self-propelled Brownian particles, where interactions between particles can give rise to various collective behaviors. This study, based on Brownian dynamics simulations, explores the effects of light intensity, rotational diffusion coefficient, and the width and spacing of illuminated regions on the aggregation structures of the system. First, this study examines the influence of light intensity on aggregation structures under different rotational diffusion coefficients, finding that as the rotational diffusion coefficient increases, the system gradually stabilizes. This stabilization is attributed to the reduced collision effects among particles at higher diffusion coefficients. Under suitable rotational diffusion coefficients, gradually increasing the ratio of longitudinal to transverse light-induced self-propulsion forces leads to a transition in the system’s aggregation structure from a transverse stripe structure configuration to a tic-tac-toe structure, ultimately resulting in a longitudinal stripe structure. This indicates that the system’s aggregation structure can be effectively controlled by changing the relative light intensity of the longitudinal and transverse illumination. From a dynamical perspective, an unstable structure consistently exhibits a super-diffusive behavior throughout the simulations, while stable structure transitions from initial super-diffusion to normal diffusion, indicating that under steady state conditions, particles aggregate in the shaded regions, exhibiting Brownian motion. To further investigate the influence of light field on collective particle behavior, in this study the width of the illuminated region and the spacing between adjacent illuminated regions are systematically varied, finding that the overall trends are consistent with previous conclusions. It is also observed that wider illumination regions with narrower spacing contribute to the formation of tic-tac-toe structures, while narrower illumination regions with wider spacing give rise to a novel structure—checkerboard structures. This study investigates the phase separation behavior of particles in complex optical field environments, providing some valuable ideas for controlling aggregation states in active particle systems.
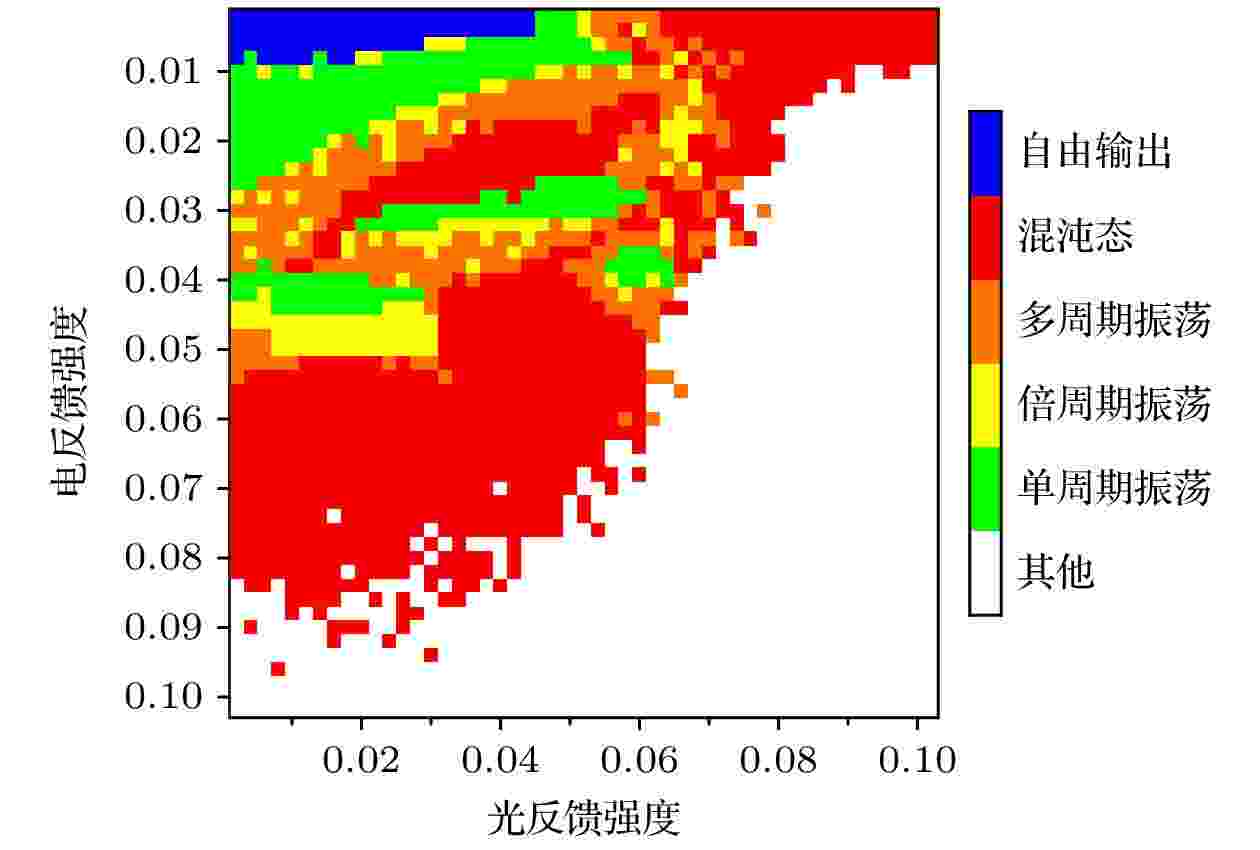
2025, 74 (9): 090502.
doi: 10.7498/aps.74.20241433
Abstract +
In this paper, various nonlinear dynamic behaviors of distributed feedback semiconductor laser (DFB-SL) subjected to self-delayed optical and electrical feedback are studied numerically. The results show that the DFB-SL output presents a variety of nonlinear dynamic states such as single-period, quasi-period, and multi-period under different optical feedback intensities. When the external light feedback reaches a certain intensity, the laser output enters a chaotic regime. When the optical feedback intensity is small, a variety of nonlinear dynamic states will appear in the DFB-SL output under different electrical feedback intensities. When the optical feedback intensity is large, the single-period dynamic state cannot be obtained by changing the electrical feedback intensity. The optical feedback and electrical feedback delay time also have a significant influence on the nonlinearity of DFB-SL. When their time delays match, the relaxation oscillation of the laser is enhanced and exhibits a single-period state. And time mismatch may lead to chaos or instability. The bias current also affects the dynamic state, however, the direction of evolution of the dynamic states is not unidirectional as the current changes unidirectionally. When the DFB-SL is in a single-period state, changing the bias current will result in the change of the single-cycle oscillation frequency. These findings provide an important theoretical basis for applying the self-delayed feedback DFB-SL to microwave photonic signal processing and secure optical communication, as well as experimental means for conducting various nonlinear scientific researches.
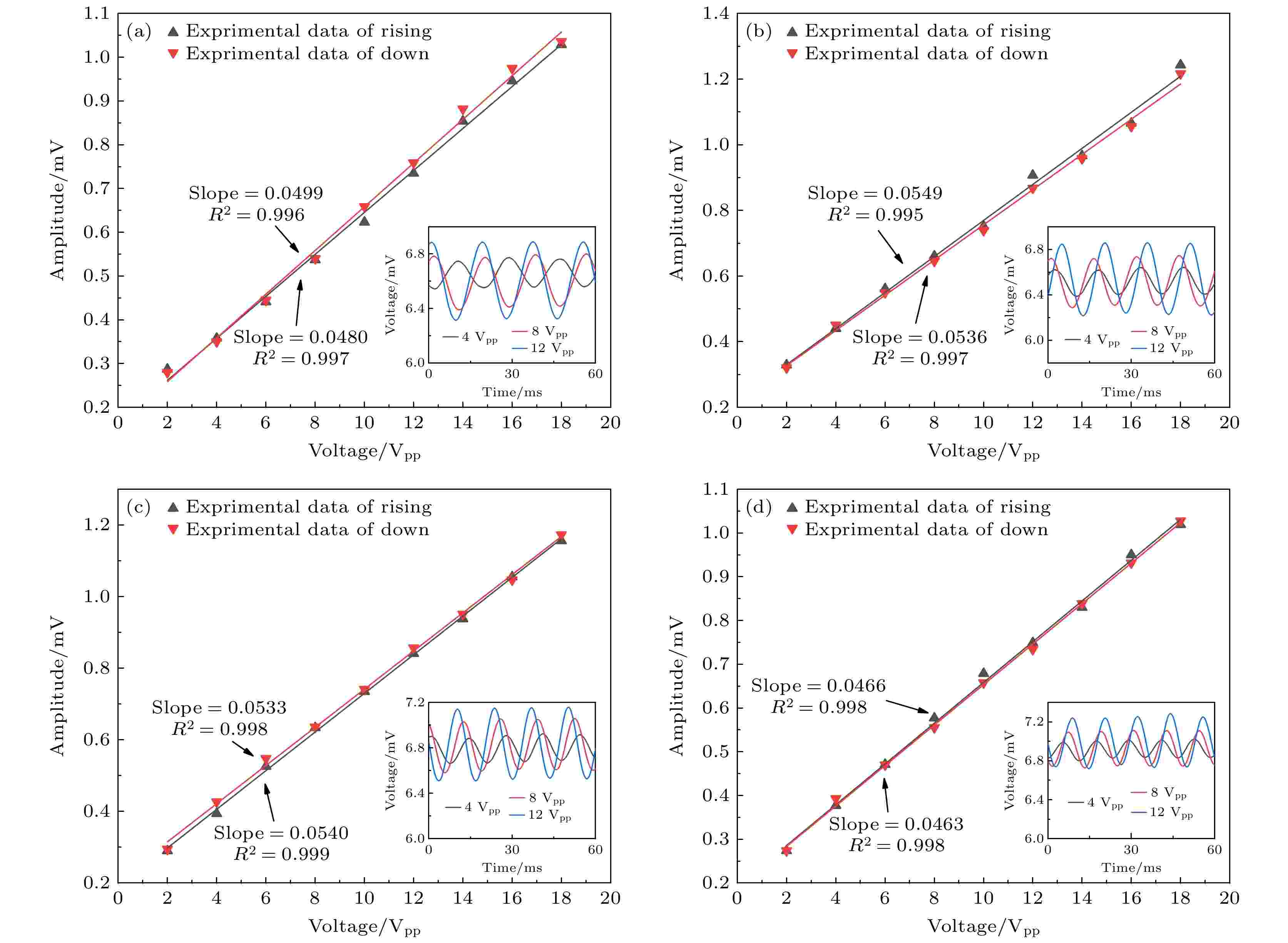
2025, 74 (9): 090701.
doi: 10.7498/aps.74.20241758
Abstract +
In this work, a low-frequency acoustic sensing scheme is proposed based on the structure of in-fiber Mach-Zehnder interferometer , in which the refractive index difference between fiber core and cladding is used to form a miniature Mach-Zehnder interferometer through fusion splicing of specialty optical fibers in a multi-mode-ultra-high numerical aperture-multi-mode configuration. This design achieves modal recombination between cladding and core modes, thereby effectively enhancing fiber bending sensitivity. The interferometer structure is then combined with a polyethylene terephthalate (PET) transducer diaphragm, enabling the sensing fiber to undergo curvature changes synchronously with the diaphragm under sound pressure, thereby indirectly increasing the area over which the fiber receives the acoustic field. When external acoustic pressure induces bending modulation on both the sensing fiber and transducer diaphragm, the differential strain distribution between the fiber cladding and core generates an optical path difference. This manifests itself in interference spectrum shifts, enabling the effective detection of low-frequency acoustic signals through demodulating the spectrum variations. In the paper, the theoretical framework for the acoustic sensing system is derived and validated experimentally. The results show that at 65 Hz, the system achieves a signal-to-noise ratio (SNR) of approximately 57 dB and a minimum detectable sound pressure of $267.9{\text{ μPa/H}}{{\text{z}}^{{{1/2}}}}$at 65 Hz. In a frequency range of 50–500 Hz, the system exhibits good acoustic response, with an SNR consistently above 40 dB and a relatively flat signal output. This scheme significantly enhances the acoustic response capability of the sensing system, enabling the effective detection of low-frequency acoustic waves. Additionally, it features simple fabrication and low cost, showing great potential for the development of acoustic wave detection applications.
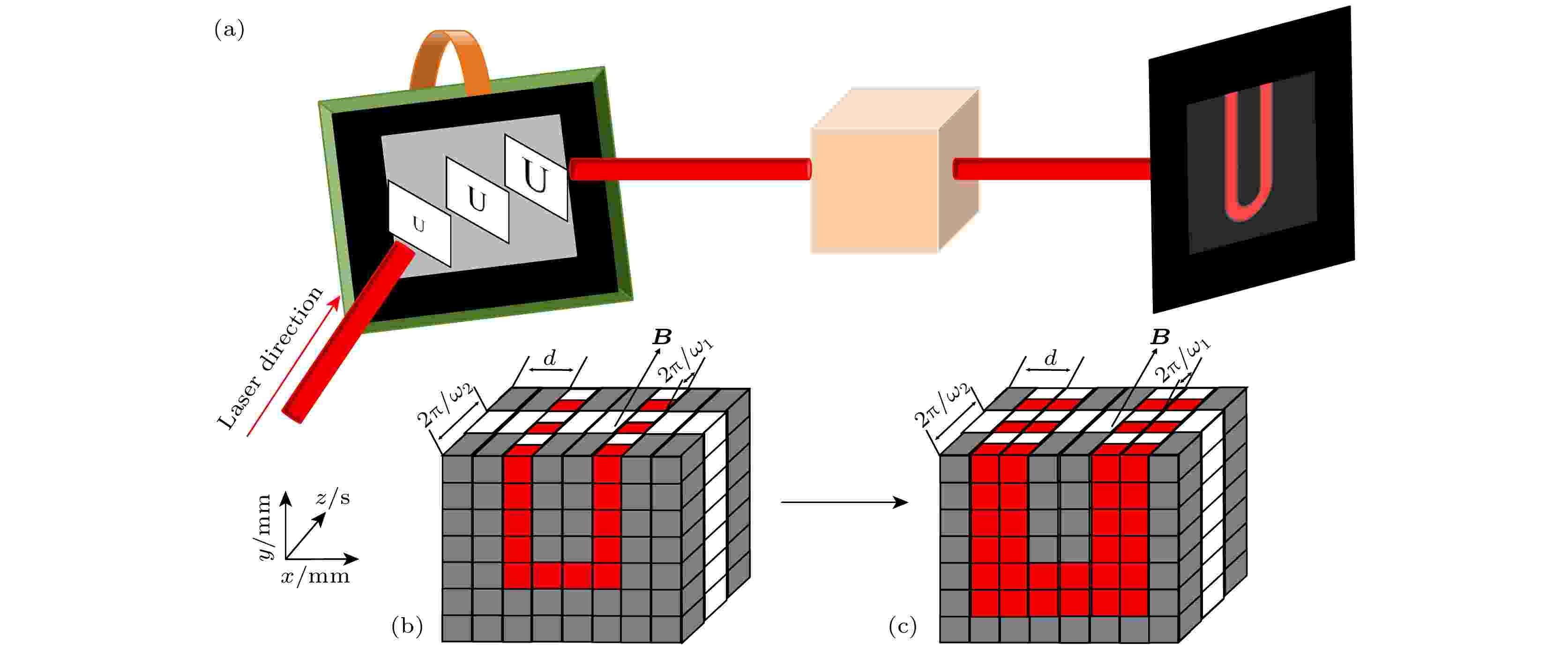
2025, 74 (9): 090702.
doi: 10.7498/aps.74.20241634
Abstract +
With the state-of-the-art quantum measurement devices, such as atomic clocks, atomic gyroscopes, and atomic magnetometers, as their central components, the spatiotemporal evolution of atomic spin polarization in the atomic vapor cell has a major effect on both increasing the bandwidth of magnetometer and improving the accuracy of magnetic gradient measurements. However, the major factor impeding the further improvement of the performance of quantum measurement instrument is the inherent static nature of the traditional intra-vapor cell segmentation imaging technique, which makes it challenging to achieve the real-time capture of the dynamic evolution of atomic spin states. In this work, we suggest a dynamic spin imaging method for alkali metal atomic vapor cells with real-time modification of atomic spin polarization states in order to overcome this technological difficulty. In particular, to ensure that the laser can precisely act on the alkali metal atoms in various regions in the vapor cell, we employ a complex beam array management system to modify the on/off state of the laser beams at various positions in the spatial dimension in real time. In the meantime, we generate laser fields with particular spatial distribution and frequency characteristics by using frequency modulation techniques in the time series to accurately regulate the on-off frequency of each laser beam in the beam array. These laser beams cause dynamic changes in the atomic spin polarization state by interacting with alkali metal atoms at various points in the vapor cell. Through precise adjustment of the laser properties, we can see and study the dynamic evolution of the atomic spin-polarization state in real time. According to the experimental data, the technology outperforms the traditional static spin imaging techniques by achieving an excellent temporal resolution of 355 frames per second and a spatial resolution of 95.9 micrometers. The effective use of this method enables us to monitor and evaluate the dynamic aspects of magnetic field distribution with unprecedented precision, also greatly enhance our understanding of the dynamic characteristics of atomic spin polarization.
NUCLEAR PHYSICS
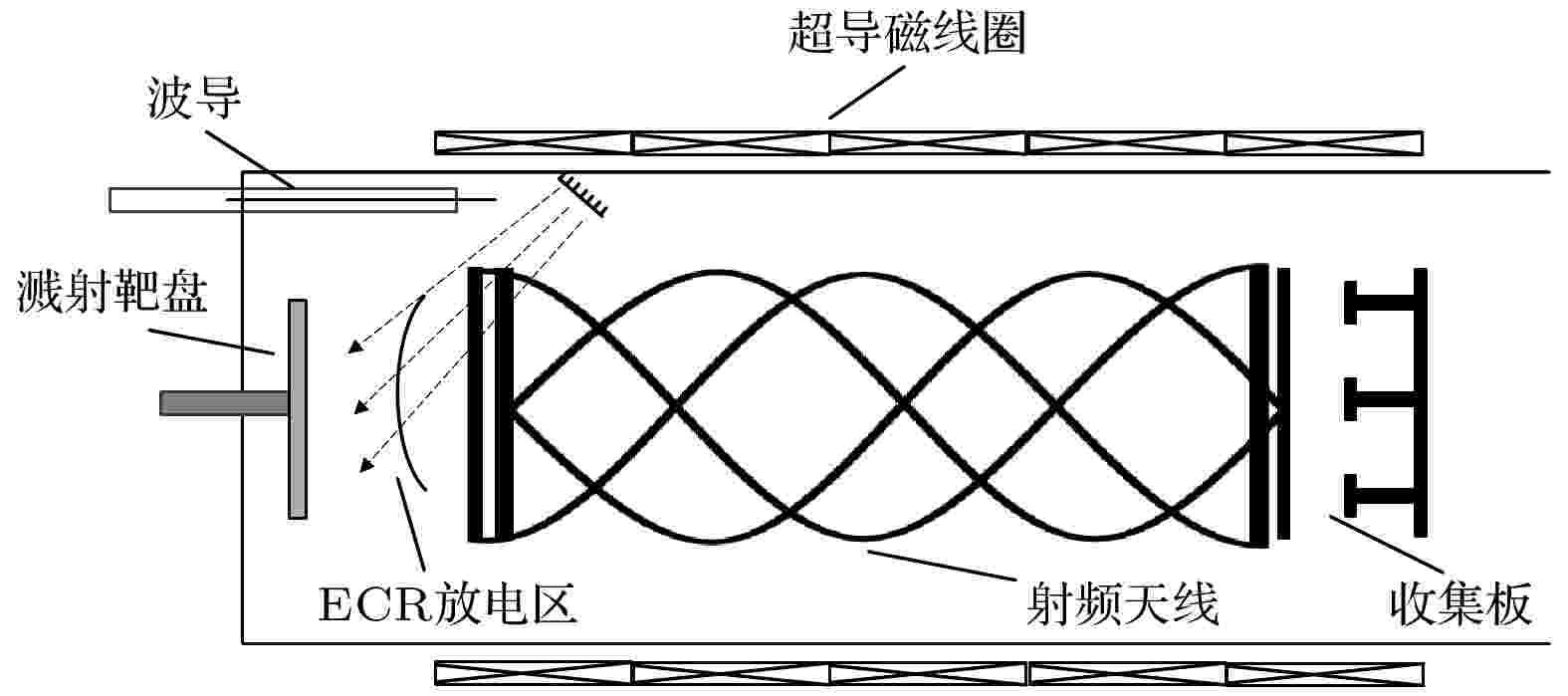
2025, 74 (9): 092801.
doi: 10.7498/aps.74.20241755
Abstract +
The ion cyclotron resonance (ICR) isotope separation method is an advanced electromagnetic separation method. The key process of this method is the transport of ions in an axial magnetic field. By injecting microwaves at the target ion cyclotron frequency, only the target ions can be heated so that the energy values of target ions can be distinguished. Due to its high separation coefficient, multiple types of isotopes that can be separated, and high flux, some countries have already built ICR isotope separation devices and conducted various isotope separation experiments since 1980. The main elements of an ICR separation device include three parts: a plasma source, a selective ion heating system, and an ion collector. The electron cyclotron resonance (ECR) ion source is the most popular plasma source, which generates the ions to be separated. The selective ion heating system is the key part of the separation device, mainly composed of a superconducting magnetic coil and a radio frequency (RF) antenna, which are used to provide a stable magnetic field and microwaves at a specific frequency to heat the target isotope ions, respectively. The ion collector is used to collect the separated ions. To clarify the key process of the ICR separation method, the transport process of ions in the electromagnetic field inside the selective ion heating system is simulated, and the influences on the selective heating effects of core parameters, such as parameters of initial plasma beam and electromagnetic field inside the selective ion heating system, are discussed in detail. The numerical simulation model used in this study is the single particle model, in which the interaction between ions and the induced electromagnetic field of the plasma beam is ignored. The simulation results show that the intensity of the alternating electric field in the selective ion heating system, the wavelength of the RF antenna, the size of the ion selective heating system, the initial axial energy of the plasma and its distribution all have a significant influence on the overall heating effect of the plasma beam. The magnetic induction intensity in the ion selective heating system, the wavelength of the RF antenna, and the initial axial energy distribution of the plasma have a direct influence on the selectivity of the heating process. Considering the limitations of the single particle model, a more accurate model will be used for further simulation. The design of the RF antenna and ECR ion source will also be considered in the further research.
ATOMIC AND MOLECULAR PHYSICS
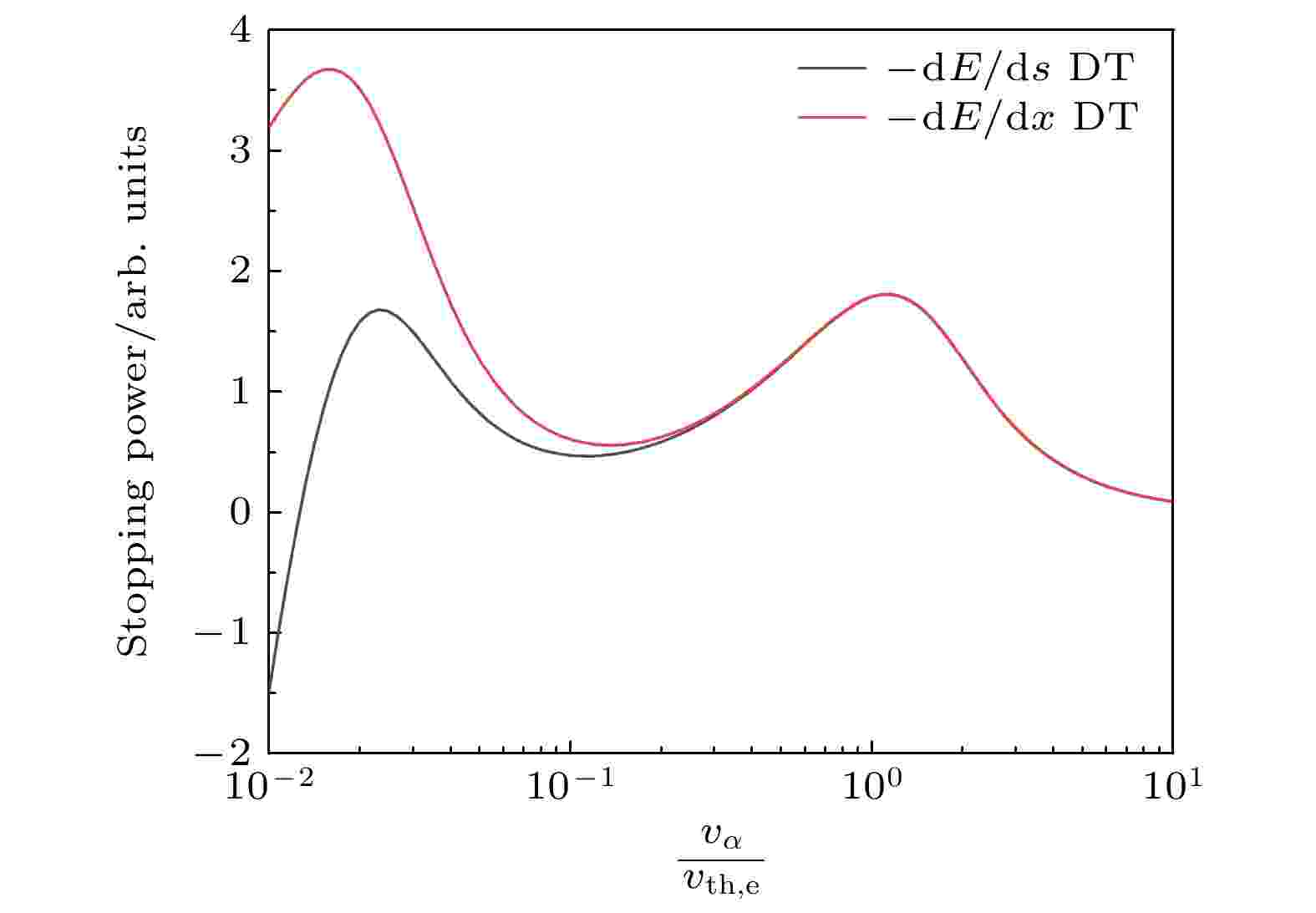
EDITOR'S SUGGESTION
2025, 74 (9): 093401.
doi: 10.7498/aps.74.20241763
Abstract +
Energy deposition of high-energy ions and the resulting electron-ion energy partition in dense plasmas are of essential importance in understanding the hot-spot ignition and burning of inertial confinement fusion. In this work, the energy deposition and electron-ion energy partition of high-energy ions are studied in a wide range of temperatures and densities based on the improved T-matrix model. Compared with the stopping power model based on the assumption of small-angle scattering, the improved T-matrix model can consistently take into account the large-angle Coulomb scattering and the resulting transversal deflection of the high-energy ions. We investigate the influence of the effect of transversal deflection on the electron-ion energy partition and propose a fitting formula for the energy partition fraction to plasma electrons. This formula is applicable to inertial confinement fusion simulations. It is found that when the effect of transversal deflection is considered, the relative energy deposited into electrons in plasma is reduced by about 27.5% at most. This conclusion suggests that the transversal deflection of energetic ions, induced by the large-angle Coulomb scattering and its cumulative effect, ought to be considered in accurately simulating the hot-spot ignition and burning of the fuel in inertial confinement fusion.
ELECTROMAGNETISM, OPTICS, ACOUSTICS, HEAT TRANSFER, CLASSICAL MECHANICS, AND FLUID DYNAMICS
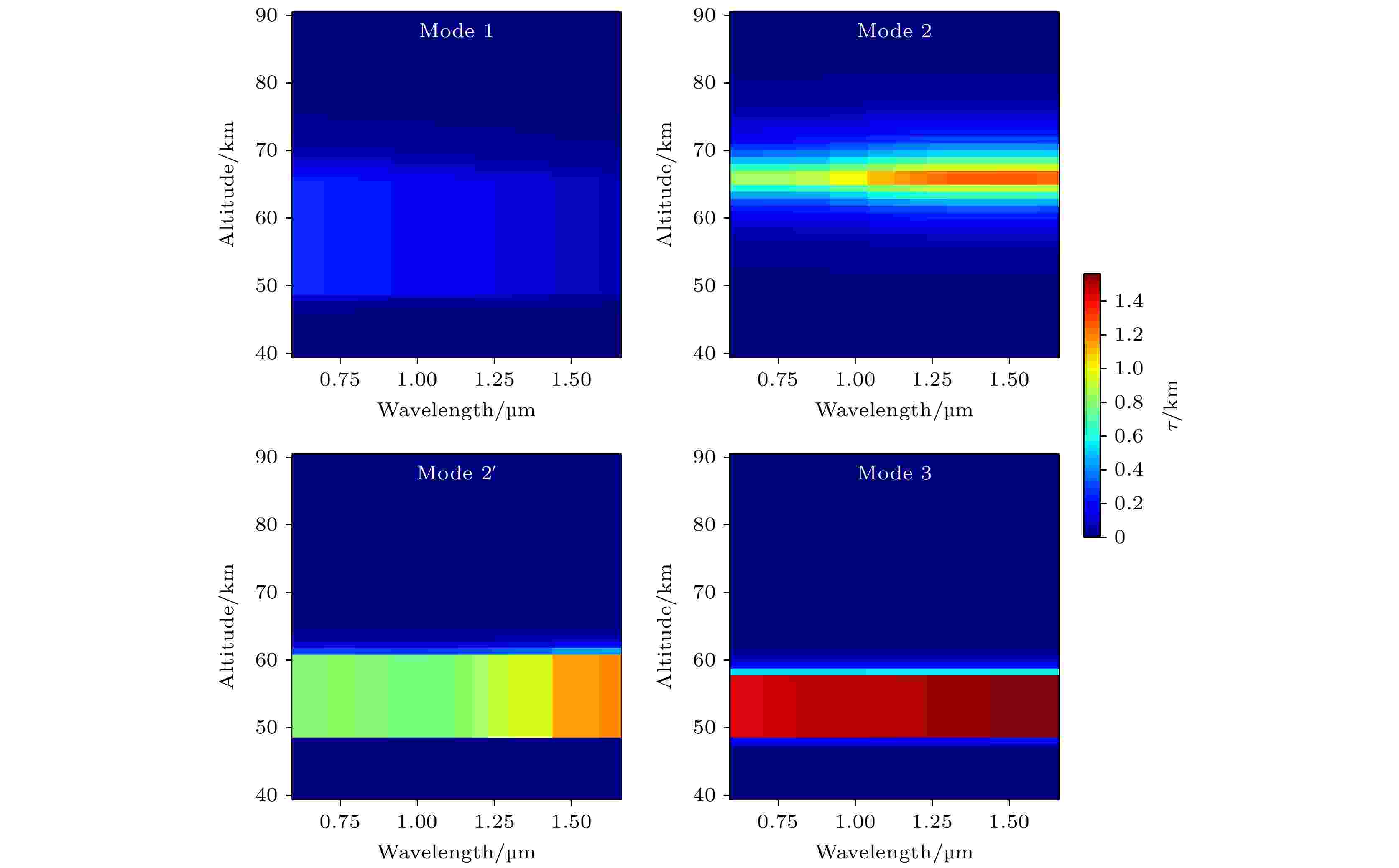
2025, 74 (9): 094201.
doi: 10.7498/aps.74.20250015
Abstract +
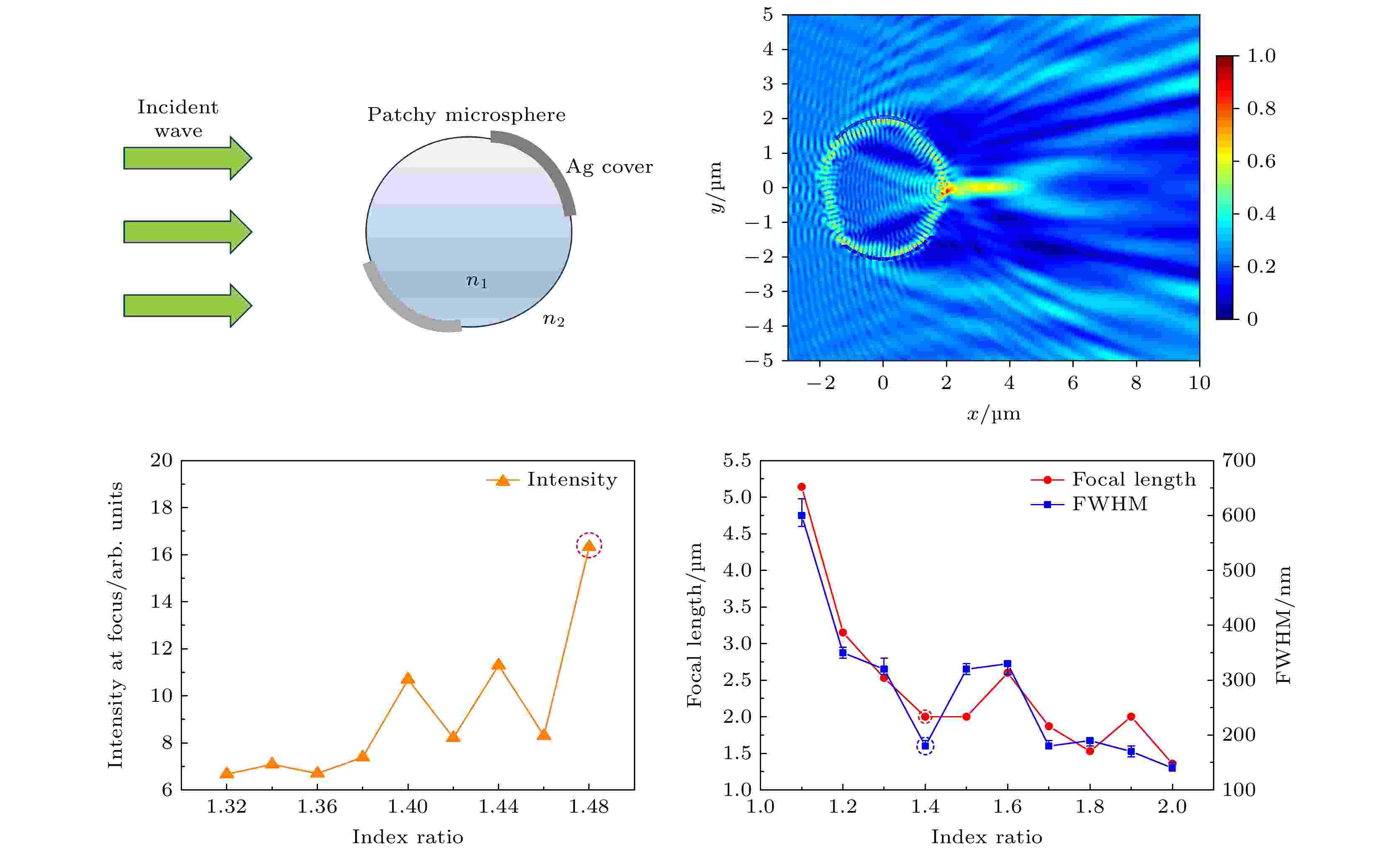
2025, 74 (9): 094202.
doi: 10.7498/aps.74.20241424
Abstract +
Photonic nanojet (PNJ) has gradually attracted the attention of researchers in the recent years. PNJ has unique properties, such as high intensity, high localization and subwavelength scale focusing ability, making it a narrow beam with wavelength scale. The full-width at half maximum (FWHM) of PNJ at the focus can exceed the diffraction limit while maintaining high intensity with a long distance, which can significantly enhance the imaging resolution. In this work, the characteristics of PNJ are explored through numerical simulation, with a focus on studying the patchy microspheres under various conditions, including coverage area, incident angle, and the refractive index of the immersion medium. The findings reveal that when the microsphere size is fixed and the coverage area accounts for 69%, the performance of PNJ is optimal. Under this condition, adjusting the incident angle to –5.74° can accurately position the PNJ focal point on the microsphere surface. Furthermore, at this specific angle, the patchy microspheres can generated PNJ with “S”-typed and “Y”-typed field intensity distribution, and the FWHM is reduced to 180 nm, significantly exceeding the traditional diffraction limit. This optimization strategy not only facilitates super-resolution focusing, but also greatly enhances both the intensity and efficiency of the PNJ. Additionally, this study demonstrates that the PNJ performance improves when the refractive index ratio between the patchy microsphere and the immersion medium approaches 1.4. Notably, a resonance effect occurs when the refractive index ratio reaches 1.48, resulting in enhanced PNJ performance. In this case, the PNJ focal point remains on the surface of the microsphere, with an FWHM of 180 nm, while the light intensity is further amplified to approximately three times the intensity of the PNJ generated by the microspheres without resonance effect. This research provides theoretical support for the application of patchy microspheres in fields such as super-resolution imaging.
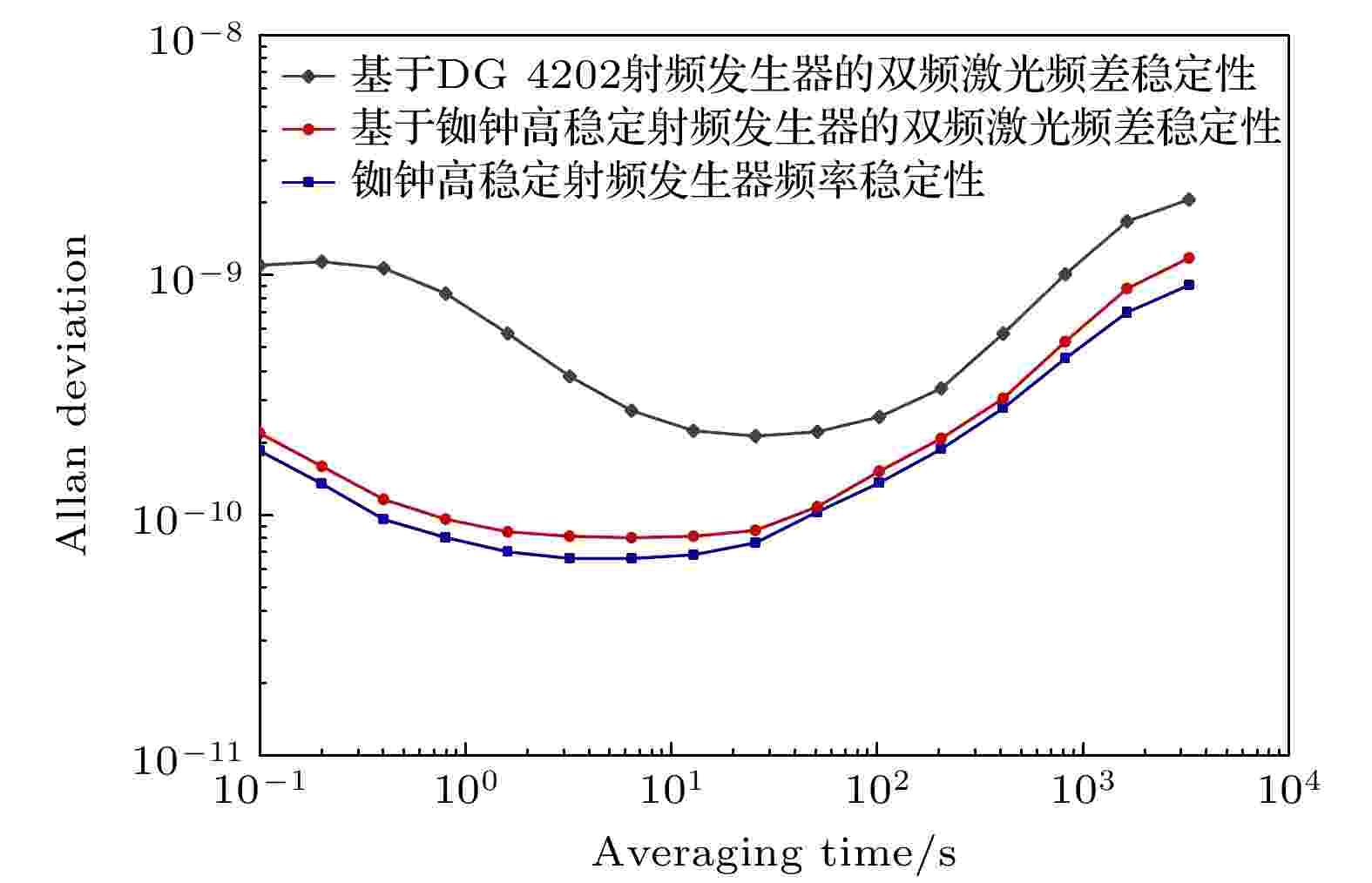
2025, 74 (9): 094203.
doi: 10.7498/aps.74.20250067
Abstract +
A high-stability dual-frequency laser source is a key technology for achieving national ultra-precision measurement capability and also the foundation for supporting the quality of high-end equipment manufacturing. In this work, a high-stability dual-frequency laser source and its frequency difference stability evaluation system are both built based on a double acousto-optic modulation scheme. By investigating the mechanism of generating dual-frequency laser based on double acousto-optic modulation, a degradation model of frequency difference stability is constructed, and targeted technical improvements are implemented. The study shows that the frequency stability of the dual-frequency laser source and the stability of the frequency difference both affect the accuracy of heterodyne interference measurement. The frequency difference stability is determined by factors such as the stability of RF signal and the nonlinear distortion of the power amplifier. This study first optimizes the frequency difference stability to 7.5×10–10@1 s and 1.2×10–9@1000 s by designing a high-order harmonic filtering technique. Then, the DG 4202 RF generator is replaced with a rubidium-clock-based high-stability RF signal generator, thus further optimizing the frequency difference stability to 9×10–11@1 s and 6×10–10@1000 s. The influence of dual-frequency frequency difference stability on heterodyne interference measurement accuracy is reduced to the sub-femtometer level. And the frequency difference stability of the dual-frequency laser source fully meets the application requirements of picometer-level laser interference measurement. Combined with the most advanced frequency stabilization technology using ultra-stable cavity, our high-stability dual-frequency laser source can support heterodyne interference measurement with picometer or even femtometer-level accuracy, demonstrating significant potential for applications in fields such as ultra-precision measurements.
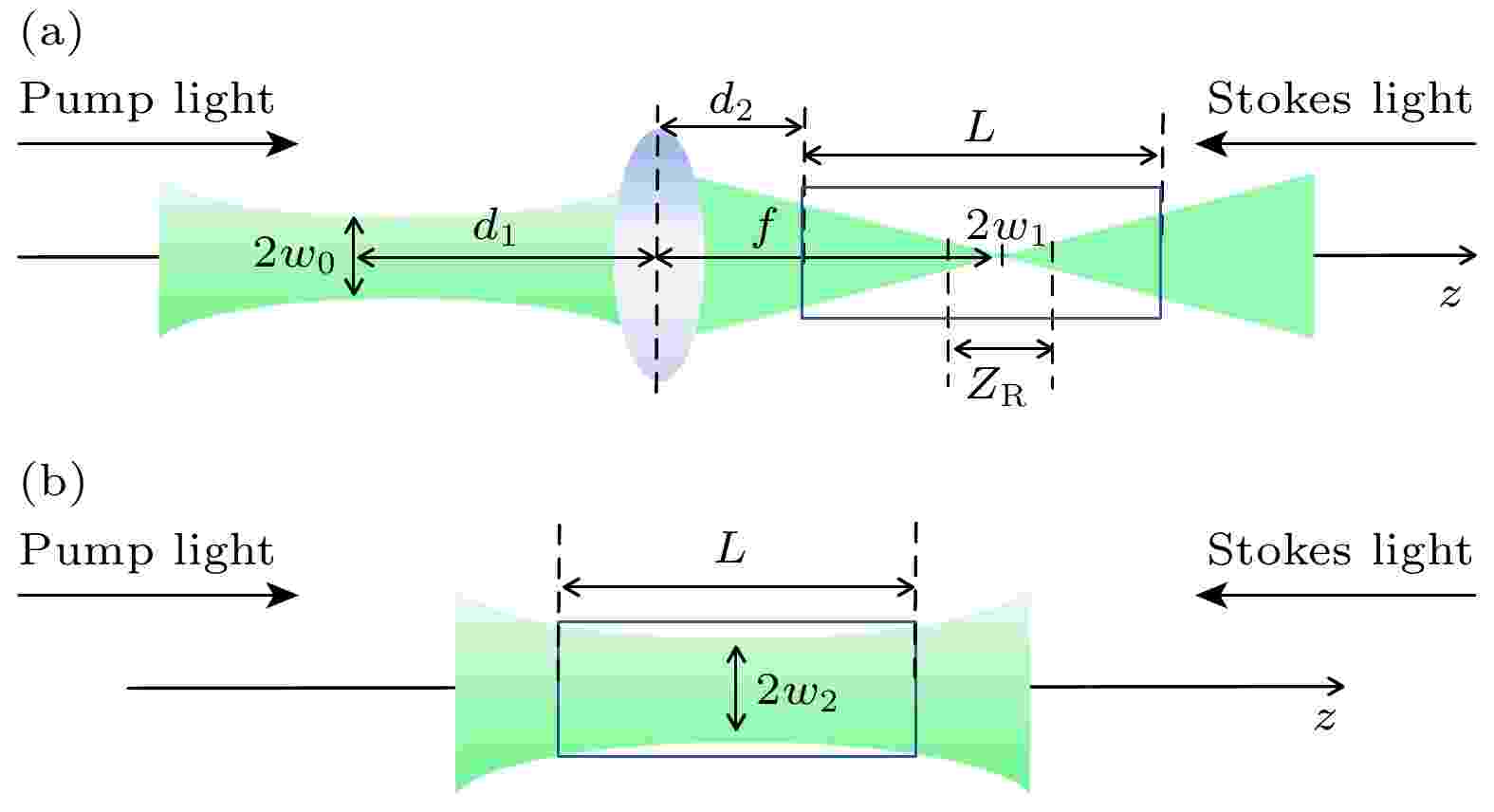
2025, 74 (9): 094205.
doi: 10.7498/aps.74.20250132
Abstract +
Stimulated Brillouin scattering lidar (SBS-LiDAR) technology possesses significant advantages such as high resolution, high signal-to-noise ratio, and strong anti-interference capacity, making it highly promising for simultaneous measurements of temperature, salinity, and sound velocity in seawater. Stimulated Brillouin scattering (SBS) is a nonlinear dynamic process characterized by temporal variations in its occurrence location, peak intensity, and spectral shape. Through numerical simulations of Stokes pulse, the conditions for SBS generation can be quantitatively determined, thereby establishing a theoretical foundation for optimizing lidar systems and enhancing their detection capabilities. Existing studies on Stokes pulses typically focus on specific experimental configurations under varying parameters, including medium properties, pump laser characteristics, and ambient environmental factors. There are still significant discrepancies in reported conclusions regarding the relationship between incident energy levels and pulse width variations, particularly in water-based environments where systematic research on the Stokes scattering pulse characteristics is clearly insufficient. In this study, a distributed noise model is used to theoretically simulate and analyze the time-domain signals of SBS in water at different laser wavelengths, pulse widths, and focal lengths. The characteristics of Stokes pulses generated by focused and non-focused configurations are investigated. The results indicate that under the same conditions, shorter incident wavelength produces significantly higher peak power of Stokes scattering light. The Stokes scattering light exhibits significant energy-dependent behavior: at low input energy, short pulse generates stronger scattering signal due to enhanced nonlinear interaction efficiency, while at high input energy, longer pulse exhibits excellent performance by maintaining temporal coherence. The larger focal length results in lower peak power but better pulse fidelity. As the incident energy increases, the pulse width of Stokes scattering light in the non-focused configuration exhibits a continuous increase. In contrast, for the focused configuration, the pulse width initially decreases and then increases, exhibiting an optimal compression value influenced by temperature and energy. At lower temperatures, the Stokes pulse width exhibits excellent compression performance near the threshold energy. Therefore, reducing secondary peak interference and suppressing spectral broadening are critical technical challenges that must be systematically addressed for short-range SBS-Lidar applications. In low-temperature detection scenarios, dynamic attenuation control becomes essential to prevent thermal stress-induced damage to photodetectors. These findings are of great significance in enhancing the performance of SBS-LiDAR system.
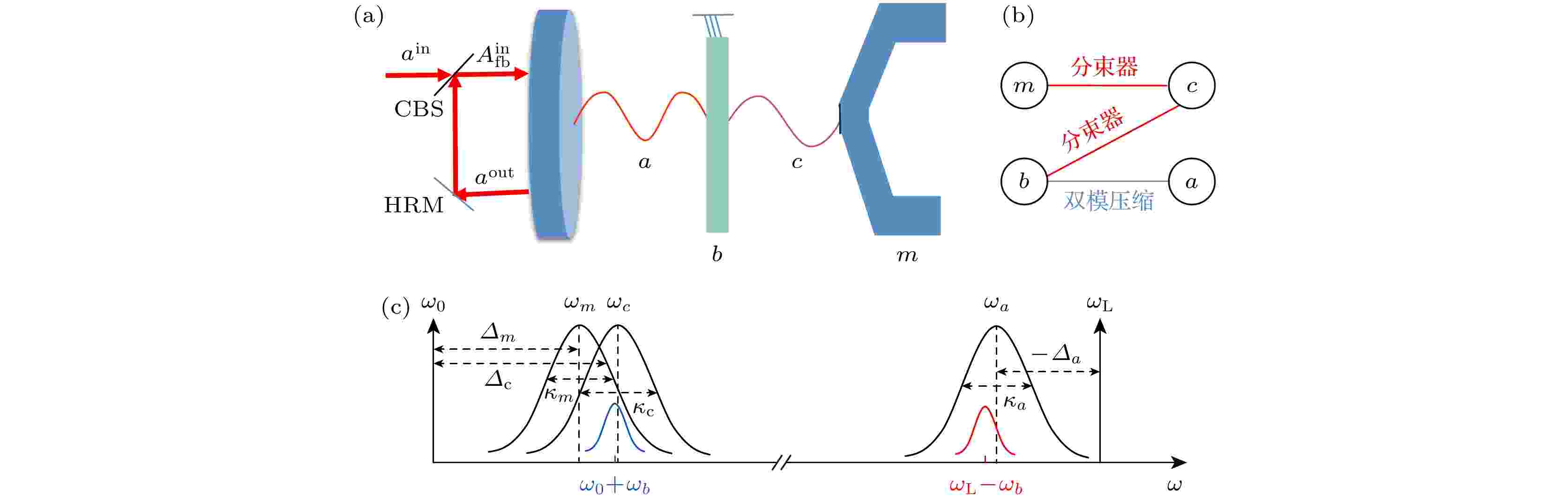
2025, 74 (9): 094206.
doi: 10.7498/aps.74.20241801
Abstract +
Optomicrowave entanglement and optomagnonic entanglement have significant applications in constructing hybrid quantum network and optical controlling magnons. In this paper, a theoretical scheme of enhancing optomicrowave and optomagnonic entanglements is proposed, based on a coherent-feedback-assisted optomagnomechanical (OMM) system. By inserting a thin membrane between the input-output mirror and the high-reflective-mirror-attached YIG bridge, the system consists of four kinds of modes: optical mode, microwave mode, mechanical mode, and magnon mode. In this system, optical mode and microwave mode interact with each other through the mechanical mode, while the magnon mode couples with the microwave mode through magnetic-dipole interaction. The entanglement is originally generated between optical mode and phonon mode under the two-mode squeezing mechanism (blue-detuned driven), then the generated entanglement is transferred to the optical mode and microwave mode through the state transfer mechanism (red-detuned driven) between the microwave mode and phonon mode and is further transferred to the optical mode and magnon mode by the magnetic-dipole interaction between the microwave mode and magnon mode. Adopting the negative logarithm criterion, the variations of the optomicrowave and optomagnonic entanglements with detuning, coupling strength, and decay rate are thoroughly investigated. Furthermore, the optimal coherent feedback parameters and the physical mechanisms of generating and transferring entanglement are analyzed, and the entanglement enhancements by adding the feedback loop are discussed. The results show that after adding coherent feedback, optomicrowave entanglement and optomagnonic entanglement can be enhanced effectively within a wide range of parameters and the enhancement can also be well maintained. Our findings provide a theoretical basis for connecting different nodes (different physical systems) to construct hybrid quantum networks, flexibly controlling the quantum properties of magnons, and preparing macroscopic quantum states.
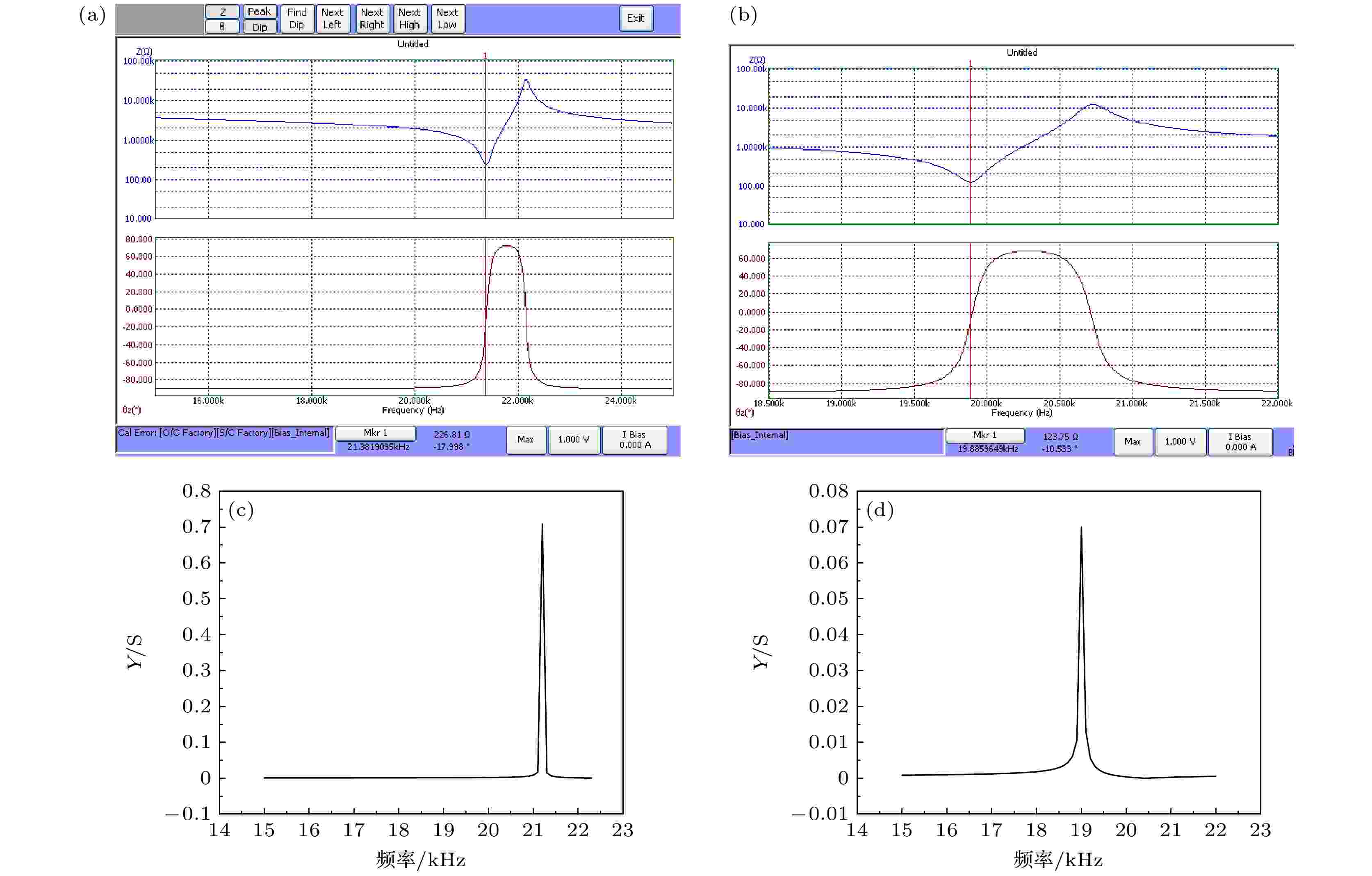
2025, 74 (9): 094301.
doi: 10.7498/aps.74.20250047
Abstract +
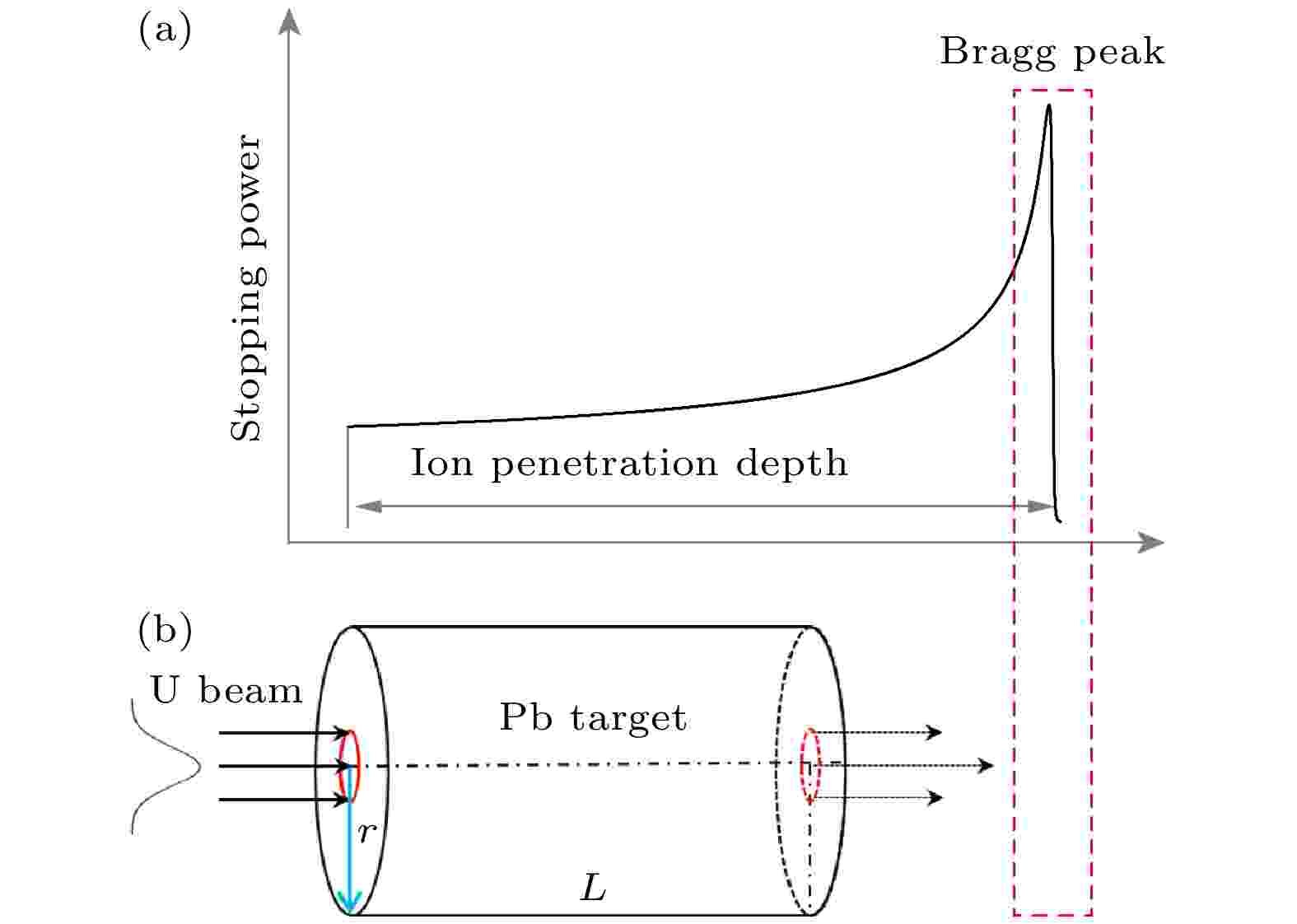
EDITOR'S SUGGESTION
2025, 74 (9): 094701.
doi: 10.7498/aps.74.20241553
Abstract +
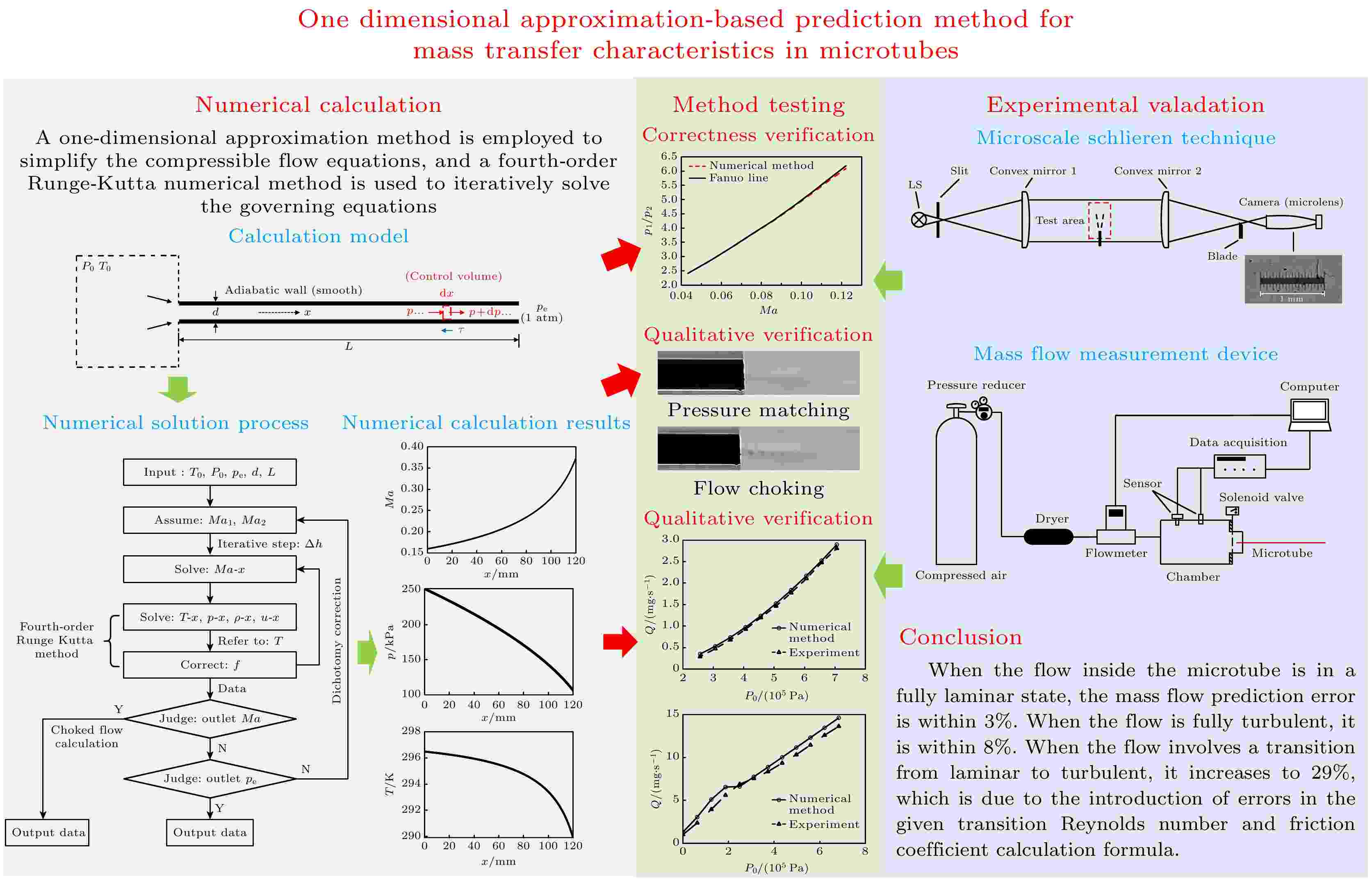
One-dimensional approximation-based method of predicting mass transfer characteristics in microtubes
2025, 74 (9): 094702.
doi: 10.7498/aps.74.20241594
Abstract +
COVER ARTICLE
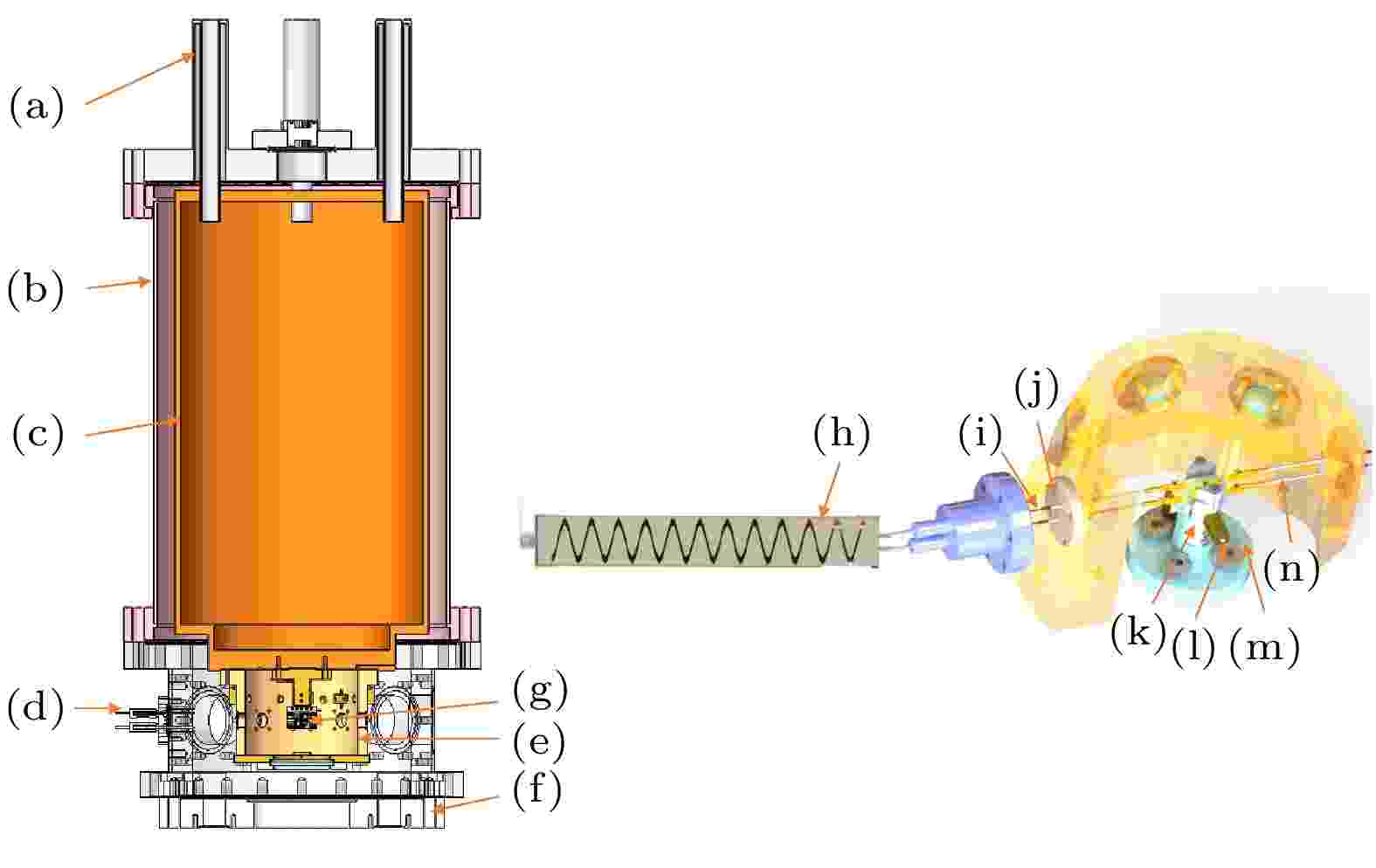
COVER ARTICLE
2025, 74 (9): 094204.
doi: 10.7498/aps.74.20250048
Abstract +
In ion optical clock systems, the motional effect of trapped ions is a key factor determining clock performance and currently representing a key limitation in achieving lower uncertainty between different ion-based optical clocks. According to the first liquid nitrogen-cooled Ca+ ion optical clock (2022 Phys. Rev. Appl. 17 034041 ), we develop a new physical system for a second Ca+ ion optical clock and make significant improvements to its ion trapping apparatus. These improvements primarily focus on two aspects. The first aspect is that we design and implement an active stabilization system for the RF voltage, which stabilizes the induced radio-frequency (RF) signal on the compensation electrodes by adjusting the amplitude of the RF source in real time. This method effectively suppresses long-term drifts in the radial secular motion frequencies to less than 1 kHz, achieving stabilized values of $\omega_x = 2\pi \times 3.522(2)\;\mathrm{MHz}$ and $\omega_y = 2\pi \times 3.386(2)\;\mathrm{MHz}$. The induced RF signal is stabilized at 59121.43(12) µV, demonstrating the high precision of the stabilization system. The second aspect is that we optimize the application of compensation voltages by directly integrating the vertical compensation electrodes into an ion trap structure. This refinement can suppress excess micromotion in all three mutually orthogonal directions to an even lower level. Tuning the RF trapping frequency close to the magic trapping condition of the clock transition, we further evaluate the excess micromotion-induced frequency shift in the optical clock to be $2(1) \times 10^{-19}$. To quantitatively assess the secular-motion of the trapped ion, we measure the sideband spectra on the radial and axial motion modes, both red and blue sideband spectra. From these measurements, we accurately determine the mean phonon number in the three motional modes after Doppler cooling, corresponding to an average ion temperature of $0.78(39)\;\mathrm{mK}$, which is close to the Doppler cooling limit. The corresponding second-order Doppler shift is evaluated to be $-(2.71 \pm 1.36) \times 10^{-18}$. The long-term stability of the radial secular motion frequency provides favorable conditions for implementing three-dimensional sideband cooling in future experiments, which will further reduce the second-order Doppler shift. These advancements not only enhance the overall stability of the optical clock but also lay the foundation for reducing its systematic uncertainty to the $10^{-19}$ level.
PHYSICS OF GASES, PLASMAS, AND ELECTRIC DISCHARGES
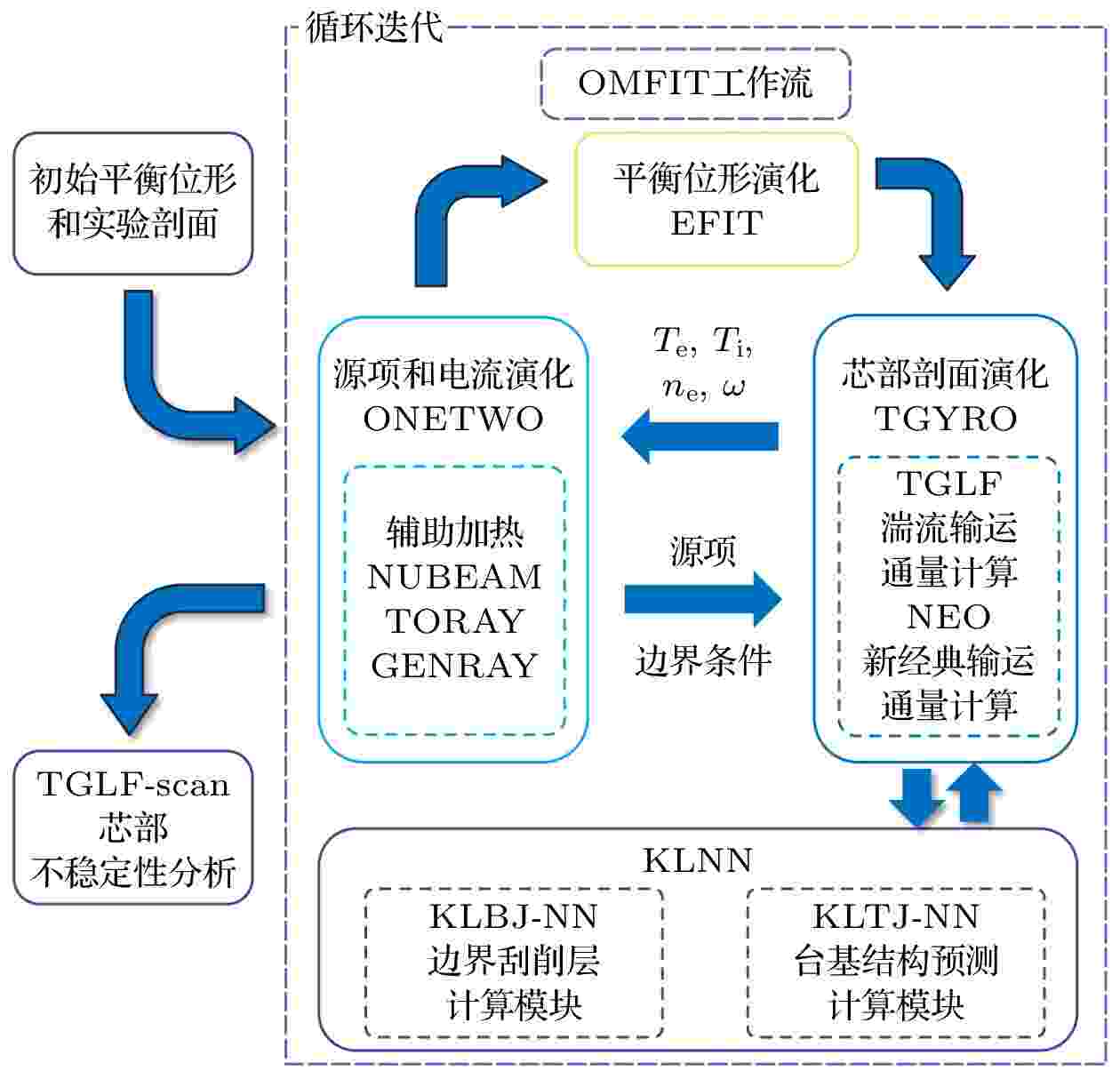
EDITOR'S SUGGESTION
2025, 74 (9): 095201.
doi: 10.7498/aps.74.20250087
Abstract +
The divertor detachment and heat flux control under high-confinement H-mode conditions in tokamaks represent critical physical challenges in current magnetic confinement fusion research. Understanding the influence of detachment on H-mode boundary transport physics, particularly its compatibility with core confinement, is central to resolving divertor detachment physics. In this study, experimental results on divertor detachment and core confinement compatibility in H-mode plasma from the HL-2A tokamak are presented. On the objective magnetohydrodynamic framework for integrated tasks (OMFIT) integrated modeling platform, a novel neural network-based fast integrated modeling method for the divertor target region is developed by integrating a new edge neural network module (Kun-Lun Neural Networks, KLNN) to enhance divertor, scrape-off-layer and edge pedestal fast prediction capability. For the first time, this method is used to conduct integrated simulations of divertor detachment and core confinement compatibility in HL-2A discharge #39007 under high-confinement mode. The simulation results are validated with experimental measurements, demonstrating that they are well consistent. Further analysis reveals that in HL-2A H-mode detachment scenarios, turbulent transport in the core region ($ 0.1 < \rho \leqslant 0.5 $) with high poloidal wave numbers $ ({k}_{\theta }{\rho }_{{\mathrm{s}}} > 1 $) is dominated by ion temperature gradient (ITG) mode, while electron-driven turbulence prevails in the region $ (0.5 < \rho \leqslant 0.7) $. In the boundary region, electron turbulence dominates at low normalized poloidal wave numbers ($ {k}_{\theta }{\rho }_{{\mathrm{s}}} < 2 $), whereas ITG modes become predominant at higher wave numbers ($ {k}_{\theta }{\rho }_{{\mathrm{s}}} > 2 $), accompanied by minor electron turbulence contributions. The research results of this work provide a certain foundation for integrated simulation and experimental verification in the study of core-edge coupling physics in tokamak devices and some insights for understanding detachment-compatible H-mode scenarios in the next-step fusion devices.
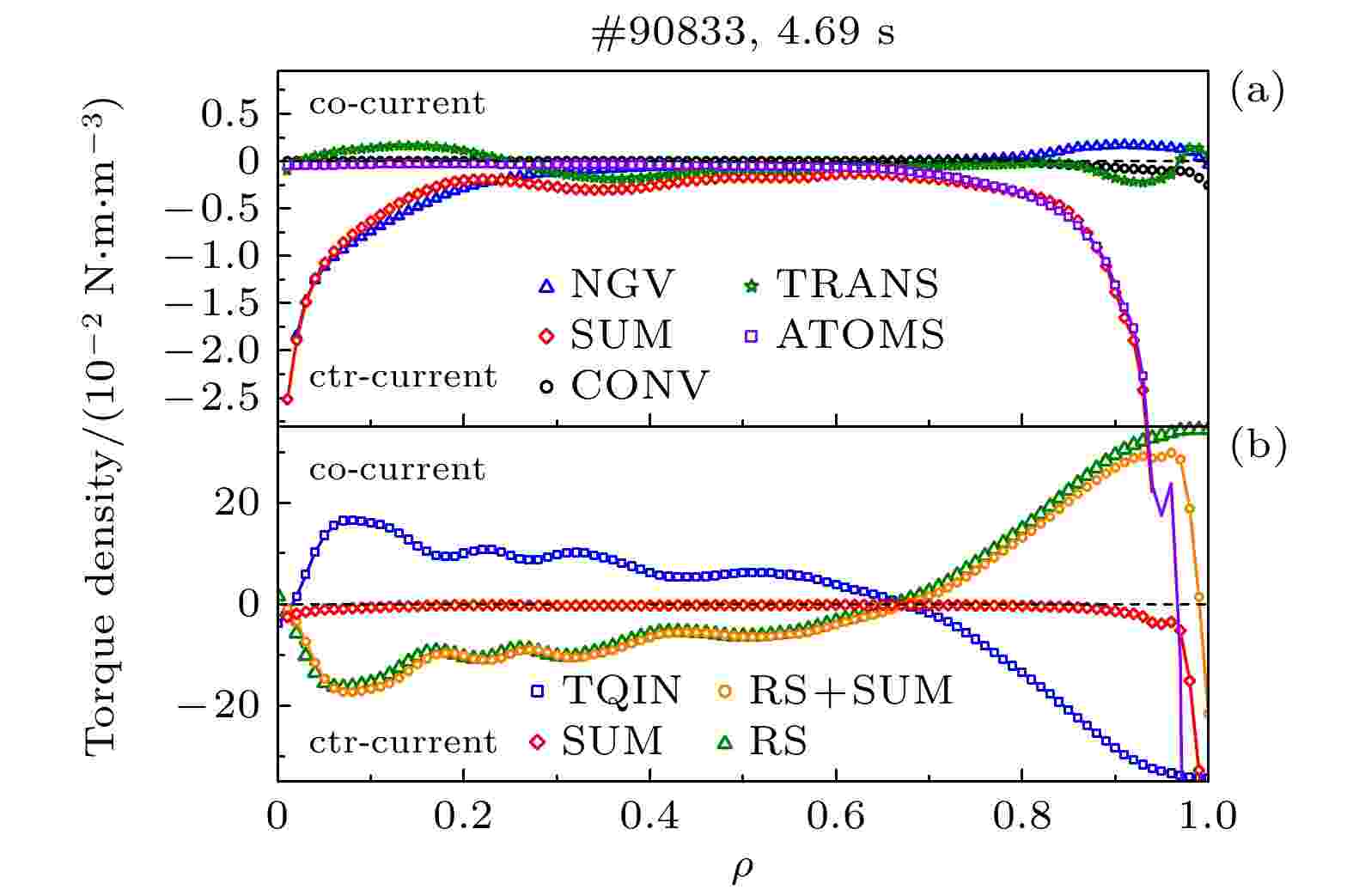
EDITOR'S SUGGESTION
2025, 74 (9): 095203.
doi: 10.7498/aps.74.20241462
Abstract +
Plasma rotation and its shear are key parameters influencing the performance of fusion devices. The prediction and control of plasma rotation velocity are of great significance for improving the stable operation and confinement of future fusion reactors. External momentum injection methods are insufficient to suppress resistive wall mode instability while achieving Q greater than 5 in international thermonuclear experimental reactor (ITER). Therefore, it is necessary to conduct experimental research on intrinsic plasma rotation that does not rely on external momentum injection. To better predict the magnitude of intrinsic rotation velocity in future fusion devices, we conduct an experimental study on the scaling of residual stress and dimensionless parameters on EAST. Using the balanced neutral beam, multiple measurements of intrinsic torque are performed, providing experimental basis for predicting the intrinsic rotation in future tokamak devices. The scaling results indicate that the core residual stress is dependent on $\rho_{\ast}^{-1.80\pm1.26}$, while the scaling of edge residual stress shows an opposite trend with $\rho _{\ast }^{1.26\pm0.63}$. This suggests that as the device size increases, the core residual stress in future large devices can increase, while the edge residual stress can decrease. The difference in scaling results between the core and edge residual stress indicates that in the edge region, the symmetry-breaking mechanism other than $\boldsymbol{E}\times\boldsymbol{B}$ flow shear dominates the generation of residual stress in the scrape-off layer. A relationship is found between intrinsic torque and $\nu _{\ast }$, revealing that the core intrinsic torque depends on $\nu _{\ast }^{-0.21\pm0.18}$. Combining the scaling results of core intrinsic torque with the gyroradius and normalized collisionality, the scaling law for core intrinsic torque is obtained to be $\rho _{\ast }^{-1.39\pm0.71}\nu _{\ast }^{0.11\pm0.10}$. Using plasma parameters of ITER operation scenario 1, the core intrinsic torque in future ITER plasma is predicted to be $(1.0\pm6.3)~{\mathrm{N}}{\cdot} {\mathrm{m}}$, which is much smaller than the predicted magnitude at DIII-D.
CONDENSED MATTER: STRUCTURAL, MECHANICAL, AND THERMAL PROPERTIES
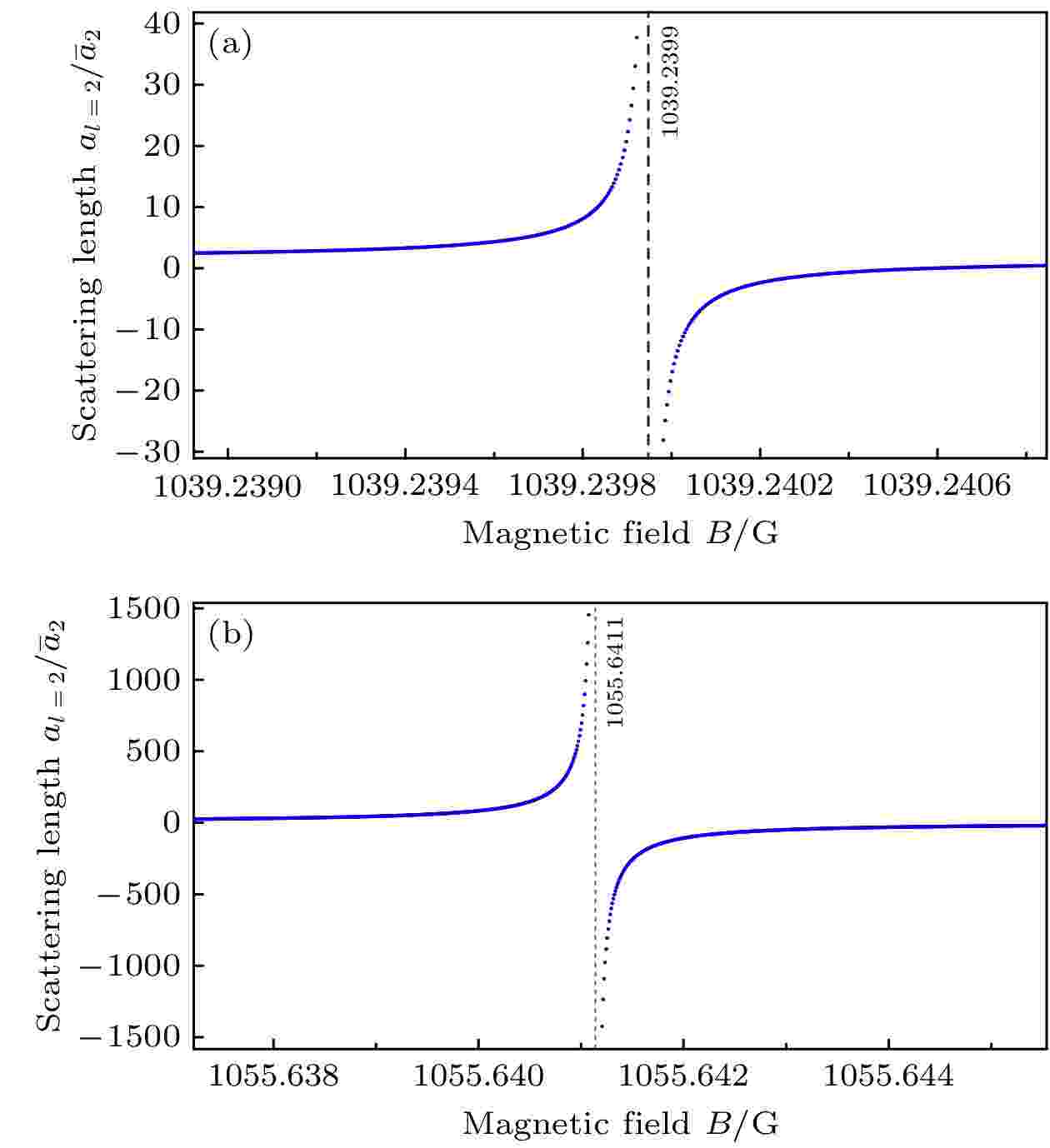
EDITOR'S SUGGESTION
2025, 74 (9): 096101.
doi: 10.7498/aps.74.20241602
Abstract +
Feshbach resonance is a fundamental phenomenon in cold atomic physics, where interatomic interactions can be precisely changed into a scattering resonance by varying an external magnetic field. This effect plays a crucial role in ultracold atomic experiments, enabling the control of interaction strength, the formation of molecular bound states, and the realization of strongly correlated quantum systems. With the rapid development of cold atom experiments, numerous Feshbach resonances corresponding to different partial waves, such as s-wave, p-wave, and even higher partial wave ones, have been experimentally identified. While s-wave resonances have been widely utilized due to their isotropic nature and strong coupling, and higher partial-wave resonances (including p-wave and d-wave resonances), provide unique opportunities for exploring anisotropic interactions and novel quantum phases. In this study, by using the multichannel quantum defect theory (MQDT), we predict that two d-wave Feshbach resonances are existent in 7Li at 1039.24 G and 1055.64 G, repectively. Physical properties of the two resonances are presented, such as the resonance width and closed channel dimer energy. In addition, we optimize the computational parameters by using the Nelder-Mead algorithm and investigate the possible resonance splitting induced by dipole-dipole interactions in higher partial waves. The presence of these d-wave resonances at high magnetic fields provides a new platform for investigating the interplay between higher-order partial wave interactions and quantum many-body effects. Our results provide opportunities for investigating the effects of higher partial wave Feshbach resonances in high magnetic fields. Our theoretical predictions thus serve as a useful reference for future experimental studies of higher-order resonance phenomena in lithium and other atomic species.
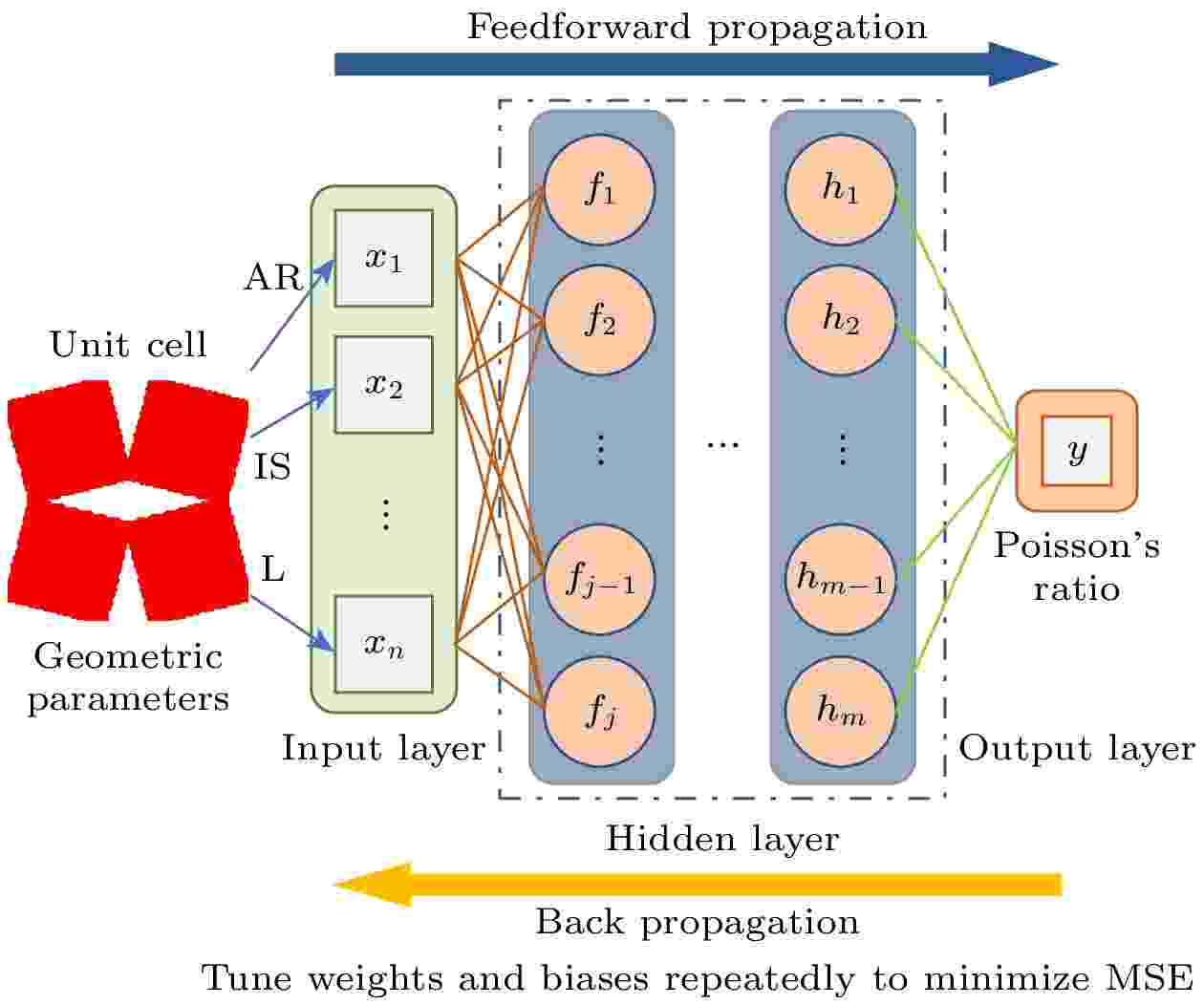
EDITOR'S SUGGESTION
2025, 74 (9): 096201.
doi: 10.7498/aps.74.20241624
Abstract +
Tuning graphene’s properties through structural design has received significant attention. However, the complex nonlinear relationship between geometric parameters of structural design and performance needs further exploring to accurately predict the performance of graphene and speed up the optimization of its structural design. This study introduces periodic rhombic perforations to effectively achieve the structural design of graphene with negative Poisson’s ratio (NPR). The mechanisms underlying the NPR effect are analyzed, and a data-driven machine learning model based on a backpropagation neural network (BPNN) is developed to efficiently predict and design perforated graphene structures exhibiting NPR. By constructing a Poisson’s ratio dataset for rhombic perforated graphene structures through molecular dynamics simulations and employing an optimized BPNN model for predictive analysis, it is found that the perforation spacing ratio (IS) has the most significant effect on the Poisson’s ratio of rhombic perforated graphene, while the perforation aspect ratio (AR) and unit cell size (L) have relatively weak influence. The study further investigates the influence of various perforation geometric parameters on the NPR behavior of graphene. It is found that reducing IS and increasing AR can enhance the negative Poisson’s ratio effect. The machine learning predictions closely align with molecular dynamics simulation results, demonstrating the effectiveness and reliability of this approach for Poisson’s ratio prediction. By integrating rhombic perforation design with machine learning technologies, this research provides an efficient framework for optimizing the NPR effect in graphene, and theoretical support for its application in smart materials and flexible electronics.
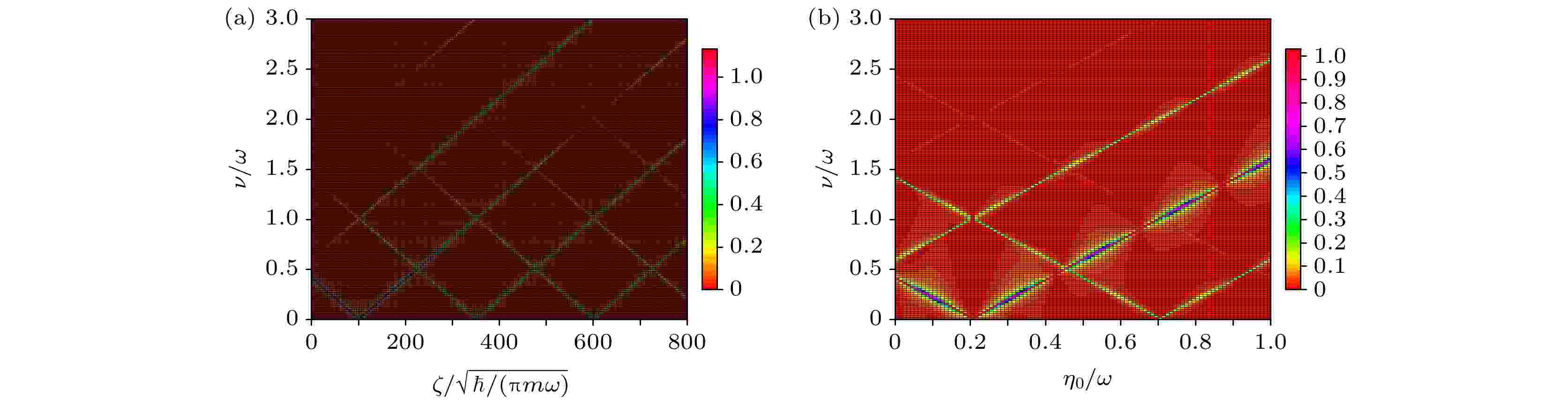
2025, 74 (9): 096701.
doi: 10.7498/aps.74.20241783
Abstract +
Dynamical characteristics of internal and external states of a Bose-Einstein condensate are generally different and independent, thus requiring different experimental manipulation techniques. The spin-orbit coupling recently achieved in Bose-Einstein condensates essentially connects spin and motion degree of freedom, endowing spin states with the ability to respond to orbital manipulation, and vice versa. In this work, a dynamical response effect, induced by simultaneously manipulating the internal and external states of a spin-orbit-coupled Bose-Einstein condensate, is predicted. Here, the “simultaneously manipulating the internal and external states” means that the driving field combines the Zeeman field applied to the internal state of the atom and the orbital potential affecting the external states of the atom. Specifically, the Bose-Einstein condensate is assumed to be activated by an abruptly applied Zeeman field and a sudden shake of the trapping potential. After some reasonable simplification and approximation of the model (i.e. neglecting the inter-atomic interactions and modelling the shake of the trapping potential by a short time-dependent pulse), an analytical relationship connecting spin frequency spectrum and the parameters of the driving fields is derived. The numerical calculations based on directly integrating the Gross-Pitaevskii equation are in good agreement with the results from the analytical relationship. The physical origin of the predicted spin dynamical response can be traced back to the quantum interference among different spin-orbit states. Due to the fact that a series of characteristic parameters of the condensate can be manifested in the spin frequency spectrum, the dynamical response effect predicted here provides a candidate method for determining and calibrating various system parameters by measuring the spin frequency spectrum.
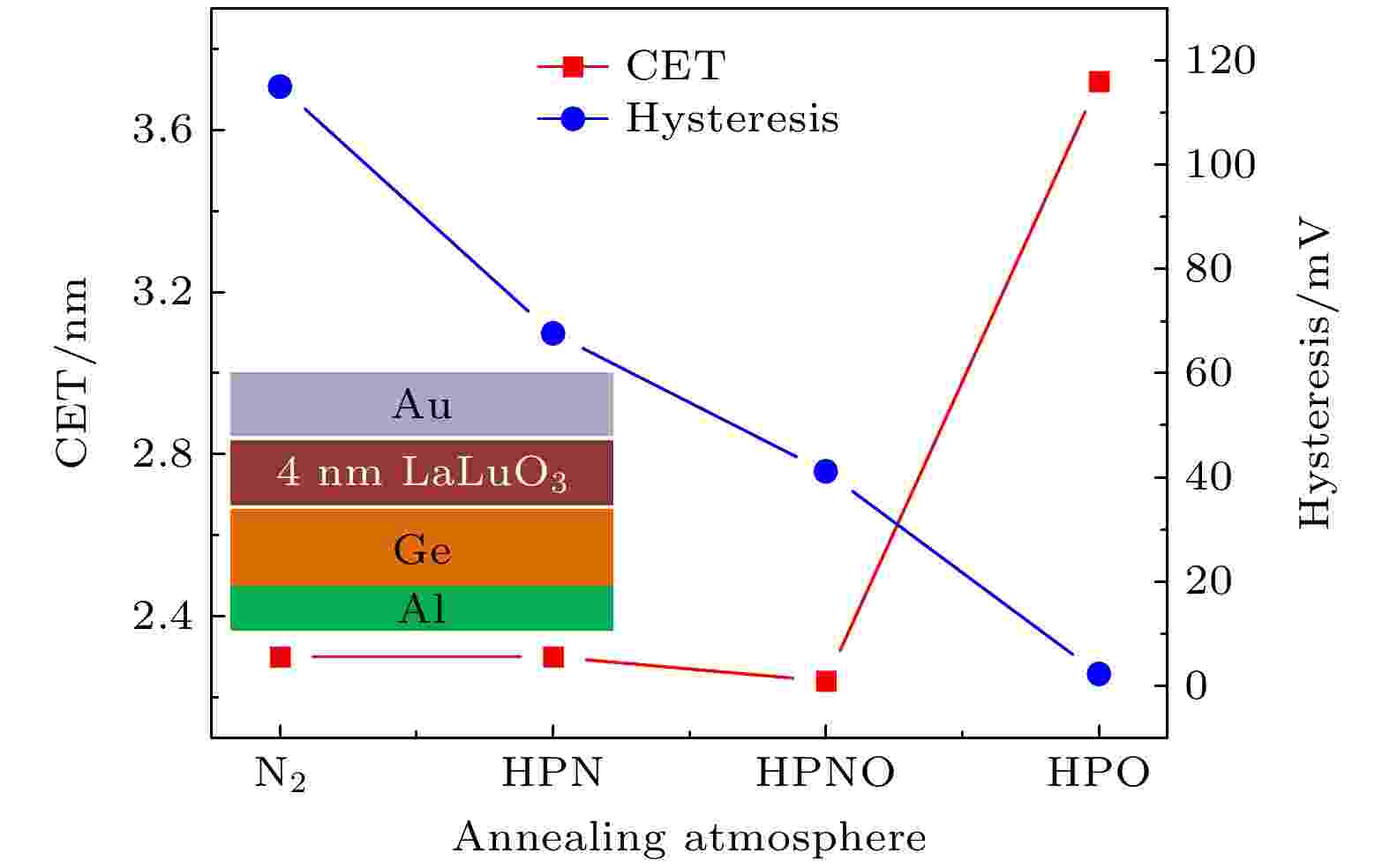
2025, 74 (9): 096801.
doi: 10.7498/aps.74.20250126
Abstract +
Germanium material holds great potential applications in low-power, high-mobility field-effect transistors because of their advantages of high electron and hole mobility, narrow bandgap, and compatibility with silicon CMOS technologies. The development of high-quality gate oxide processes is crucial in fabricating high-mobility Ge-based transistors, especially those with high dielectric constant for superior gate control and preferable gate stability. Rare-earth oxides represented by LaLuO3 have high dielectric constants and high crystallization temperatures, making them potential candidates for Ge-based metal-oxide-semiconductor field-effect transistor (MOSFET) gate technology. In this work, a germanium (Ge)-based oxide dielectric LaLuO3 is fabricated utilizing a p-type Ge substrate with a (111) crystal orientation and a doping concentration of 1×1016 cm–3, and radio-frequency (RF) co-sputtering 2-inch 99.9% La2O3 and Lu2O3 targets. Systematical investigations are conducted to evaluate the effects of annealing process conditions on the characteristics of the LaLuO3/Ge MOS gate structure under three specifically designed annealing atmospheres, i.e. nitrogen, oxygen, and a nitrogen-oxygen mixed gas with an N2:O2 ratio of 0.999∶0.001. Meanwhile, the influence of annealing pressure is also explored. The results show that annealing in pure oxygen at atmospheric pressure can reduce the hysteresis of gate capacitance, but it can lead to the formation of interface layers. Correspondingly, annealing technique based on high-pressure and low-oxygen-content (0.1% O2) atmosphere is developed, which not only improves the LaLuO3/Ge interface quality and suppresses the oxygen vacancy generation, but also achieves an extremely low equivalent oxide thickness (EOT) of 1.8 nm and a hysteresis voltage of only 40 mV, resulting in an ideal LaLuO3/Ge MOS structure. This work thus provides a high-performance LaLuO3/Ge gate process solution for Ge MOSFETs.
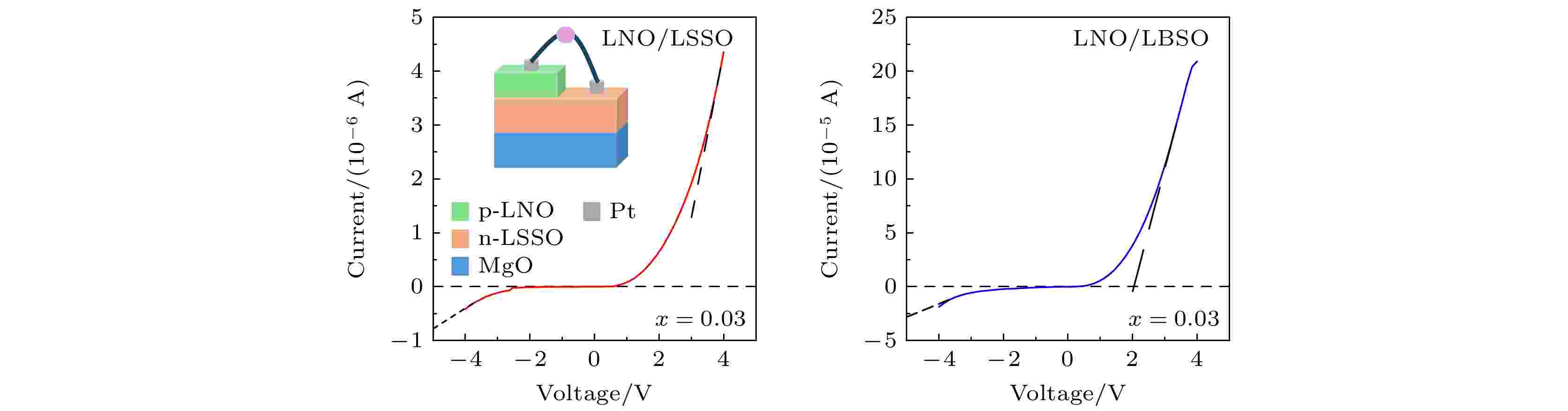
2025, 74 (9): 096802.
doi: 10.7498/aps.74.20241683
Abstract +
Transparent conducting oxides (TCOs) are crucial materials in optoelectronics, but p-type TCOs are less studied than n-type TCOs. NiO, for typical p-type TCOs show promising potential applications in transparent optoelectronic devices. In this study, LixNi1–xO thin films with varying thickness and Li doping levels on MgO(001) substrates are successfully fabricated using pulsed laser deposition. The results demonstrate that increasing both thickness and Li doping level will reduce the resistivity of the films, with the maximum optical bandgap observed at a thickness of 50 nm and 3% Li doping level. Based on the control of physical properties through film thickness and Li doping, the p-type LixNi1–xO with the largest bandgap is selected to construct transparent electronic devices with n-type La-doped ASnO3 films. The I -V tests confirm the rectification properties of the heterostructures, successfully demonstrating the formation of pn junctions. This work expands the potential applications of transparent electronic devices by integrating p-type NiO with n-type ASnO3.
CONDENSED MATTER: ELECTRONIC STRUCTURE, ELECTRICAL, MAGNETIC, AND OPTICAL PROPERTIES
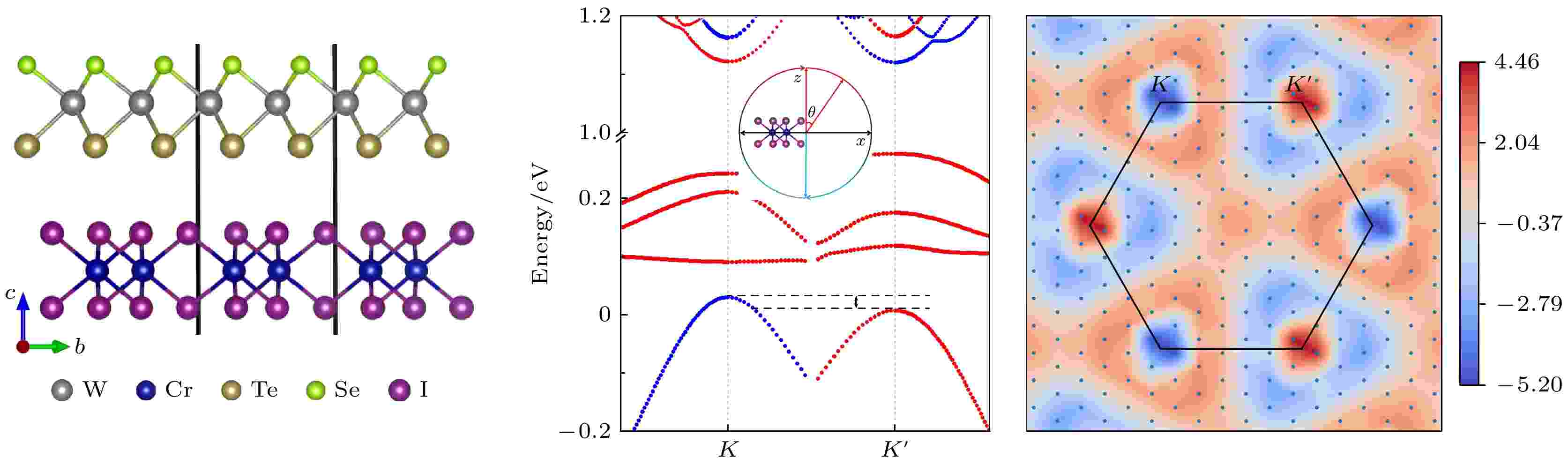
2025, 74 (9): 097101.
doi: 10.7498/aps.74.20241750
Abstract +
The valley degree of freedom, besides charge and spin, can be used to process information and perform logic operations as well, with the advantage of low power consumption and high speed. The effective manipulation of valley degrees of freedom is essential for their practical applications in valleytronics and spintronics. In this work, the effective strategy is investigated for the valley manipulation of the WSeTe/CrI3 van der Waals heterojunction with about 2% lattice mismatch by the first-principles calculations. The valley degree of freedom in WSeTe can be modulated by the magnetism of Cr atoms in the substrate via the magnetic proximity effect, including the vertical strain method and the rotation of the magnetic moments of Cr atoms. First-principles calculations are performed by using the VASP software package with the generalized gradient approximation functional in PerdewBurke-Ernzerhof (PBE) form. The spin-orbit coupling is considered when calculating the band structure to investigate the valley properties. The dependence of valley polarization on both vertical strain and the substrate’s magnetic moment direction has been systematically analyzed. There are two different stacking configurations for the WSeTe/CrI3 heterojunction with Te/Se atoms at the interface, namely Te-stacking and Se-stacking. Although single-layer WSeTe does not have valley polarization, the Te-stacked and Se-stacked WSeTe/CrI3 heterojunctions exhibit valley polarizations of 25 meV and 2 meV, respectively, which is influenced by spin-orbit coupling and the proximity effect of the magnetic substrate CrI3, indicating the importance of the stack configuration. The Te-stacked configuration of the heterojunction has a larger valley polarization due to stronger orbital hybridization between W atoms in WSeTe layer and Cr atoms in CrI3 layer. The application of vertical strain, which effectively tunes the interlayer distance, significantly regulates the valley polarization. Specifically, the valley polarization is increased to 59 meV when the interlayer distance decreases by 0.5 Å, while it decreases to 10 meV when the interlayer distance increases by 0.5 Å. Additionally, when the magnetic moment of the CrI3 substrate rotates by 360°, the valley polarization changes between –25 meV and 25 meV. It reaches a maximum value when the magnetic moment is aligned along the out-of-plane direction. This study demonstrates that the valley degree of freedom in the WSeTe/CrI3 van der Waals heterojunction can be effectively manipulated by adjusting the interlayer distance through vertical strain and by controlling the magnetic moment direction of the substrate. These findings provide valuable insights into the design and application of valleytronic and spintronic devices based on two-dimensional van der Waals heterostructures.
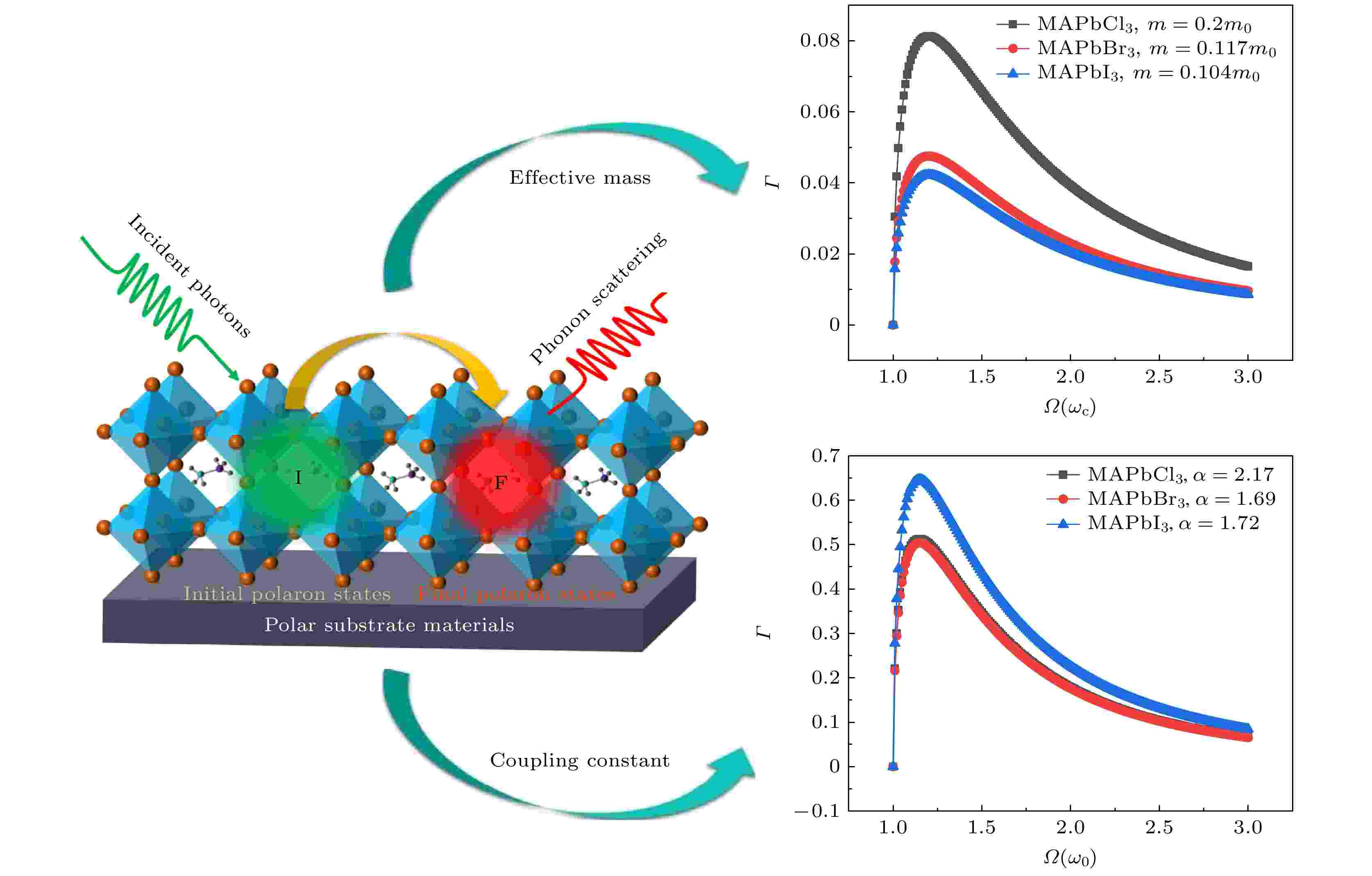
2025, 74 (9): 097102.
doi: 10.7498/aps.74.20250105
Abstract +
Perovskite quantum dots, as an emerging class of nanomaterial, have demonstrated significant potential applications in the field of optoelectronic energy conversion due to their unique optoelectronic properties. In particular, polarons play a crucial role in the optical and optoelectronic performance of perovskite quantum dots. Polaron formation, which involves the coupling of electrons with lattice phonons, can induce charge shielding effect and localization effect, thereby protecting charge carriers from scattering and recombining. This leads to longer carrier lifetimes and diffusion lengths, thereby enhancing the efficiency of optoelectronic energy conversion. In this study, a polaronic light absorption model is established using unitary transformation and the Larsen method, revealing the dependence of polaronic transition optical absorption on the electron-phonon coupling constant and effective mass in perovskite quantum dots. The results indicate that the vibration frequency, excited-state energy of polarons, and the transition spectral line frequency are closely related to the electron-phonon coupling strength and effective mass. Specifically, as the electron-phonon coupling constant increases, the vibration frequency and excited-state energy of polarons decrease, while the transition spectral line frequency increases. This finding not only elucidates the physical mechanism of polaronic optical absorption but also provides new insights and methods for optimizing the performance of perovskite quantum dot materials. Moreover, this research expands the application scope of perovskite quantum dots in fields such as photodetectors, light-emitting diodes (LEDs), and solar cells. For instance, in LEDs, the high photoluminescence quantum yield and tunable bandgap of perovskite quantum dots make them ideal luminescent materials. In solar cells, their excellent optoelectronic conversion efficiency and carrier transport properties can significantly enhance device performance. By further optimizing polaron-related characteristics, it is expected that the performance of perovskite quantum dots in these applications can be further improved.
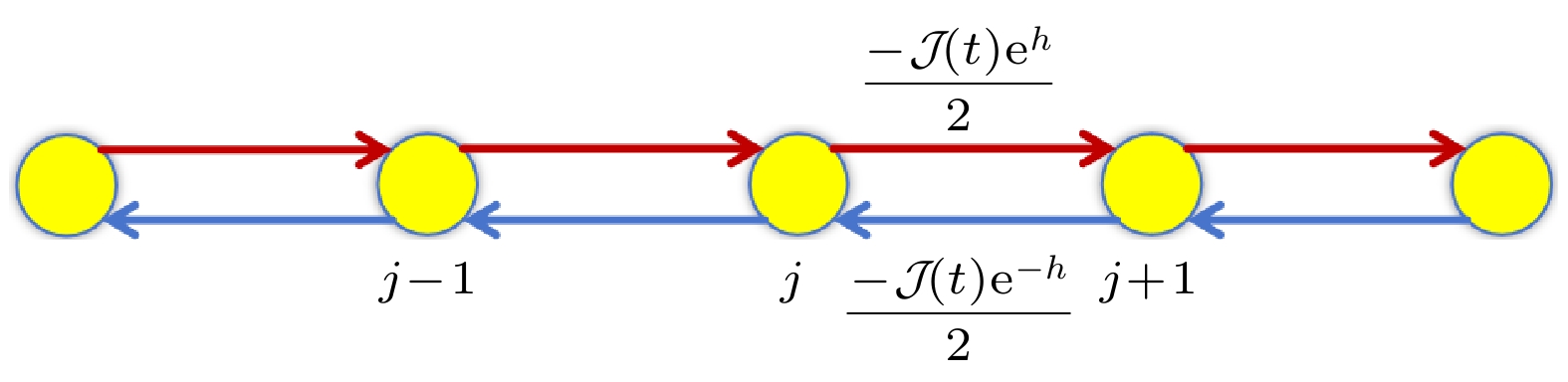
2025, 74 (9): 097201.
doi: 10.7498/aps.74.20241633
Abstract +
In this work, we investigate the delocalization-localization transition of Floquet eigenstates in a driven chain with an incommensurate Aubry-André (AA) on-site potential and a small non-reciprocal hopping term that is driven periodically in time. The driving protocol is chosen such that the Floquet Hamiltonian corresponds to a localized phase in the high-frequency limit and a delocalized phase in the low-frequency limit. By numerically calculating the inverse participation ratio and the fractal dimension $D_q$, we identify a clear delocalization-localization transition of the Floquet eigenstates at a critical frequency $\omega_{\rm c}\approx0.318\pi$. This transition aligns with the real-to-complex spectrum transition of the Floquet Hamiltonian. For the driven frequency $\omega>\omega_{\mathrm{c}}$, the system resides in a localized phase, and we observe the emergence of CAT states—linear superposition of localized single particle states—in the Floquet spectrum. These states exhibit weight distributions concentrated around a few nearby sites of the chain, forming two peaks of unequal weight due to the non-reciprocal effect, distinguishing them from the Hermitic case. In contrast, for $\omega<\omega_{\mathrm{c}}$, we identify the presence of a mobility edge over a range of driving frequencies, separating localized states (above the edge) from multifractal and extended states (below the edge). Notably, multifractal states are observed in the Floquet eigenspectrum across a broad frequency range. Importantly, we highlight that the non-driven, non-reciprocal AA model does not support multifractal states nor a mobility edge in its spectrum. Thus, our findings reveal unique dynamical signatures that do not exist in the non-driven non-Hermitian scenario, providing a fresh perspective on the localization properties of periodically driven systems. Finally, we provide a possible circuit experiment scheme for the periodically driven non-reciprocal AA model. In the following work, we will extend our research to clean systems, such as Stark models, to explore the influence of periodic driving on their localization properties.
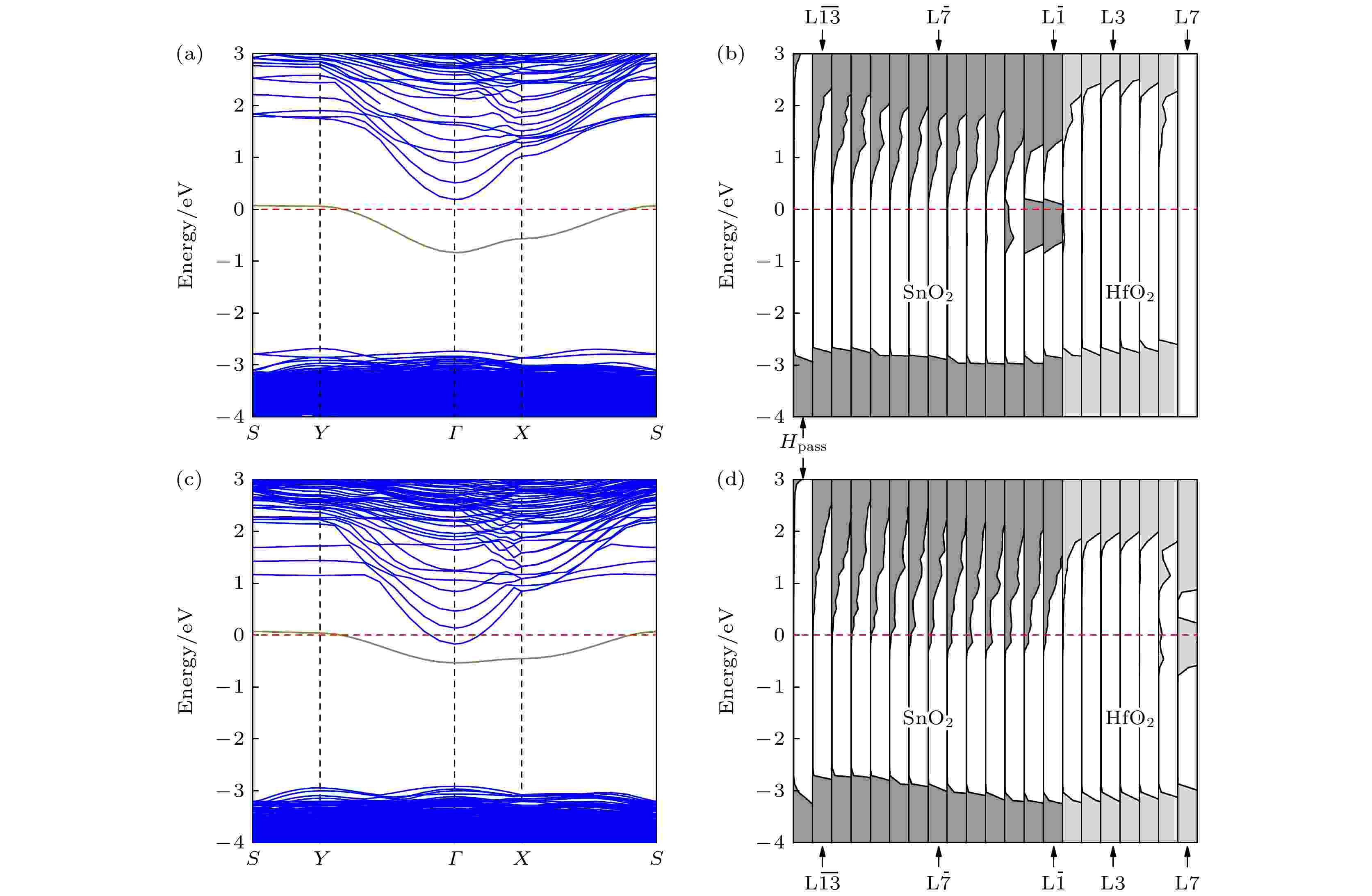
2025, 74 (9): 097301.
doi: 10.7498/aps.74.20250114
Abstract +
In SrTiO3-based oxide heterostructures, the mobility of the two-dimensional electron gas (2DEG) at the interface is relatively low at room temperature due to the influence of Ti 3d orbitals, which limits their applications in semiconductor devices. In contrast, the conduction band bottom of SnO2 is composed of Sn 5s orbitals, and it has been demonstrated that bulk SnO2 exhibits high carrier mobility at room temperature. Therefore, SnO2-based heterostructure interfaces have the potential to form 2DEG with high mobility at room temperature. In this paper, we construct a heterostructure (HfO2)7/(SnO2)13 with $2 \times 1$ supercell in (001) plane and systematically investigate the electronic structure of the heterostructure by using first-principles calculations. The calculation results show that the defect-free (HfO2)7/(SnO2)13 heterostructure has a band structure similar to that of a semiconductor, and there is no 2DEG near the interface of the heterostructure. However, the conduction band bottom is mainly contributed by non-degenerate Sn 5s orbitals in this situation. In the in-plane $2 \times 1$ supercell of the (HfO2)7/(SnO2)13 heterostructure, each layer contains 8 oxygen atoms (the thickness of 1 unit cell is defined as a layer). When an oxygen atom in a layer on the SnO2 side near the interface of the heterostructure is removed, the presence of the oxygen vacancy leads to the formation of a defect band below the conduction band. This will lead to hopping conductivity in the heterostructure. However, 2DEG still does not appear near the heterostructure interface. When the oxygen vacancy is located in the surface layer of the HfO2 in the supercell structure, the presence of the oxygen vacancy leads to the formation of a defect state in the surface. The electrons in the defect state are localized and do not contribute to conductivity. However, the defect band overlaps with the conduction band at the interface, causing the electrons on the surface of HfO2 to tunnel towards the interface. In this scenario, the 2DEG emerges in the vicinity of the heterostructure interface. In addition, for HfO2/SnO2 heterostructures with thinner HfO2 layers, such as HfO2 layer with a thickness of 7 unit cells (about 2.37 nm), the H atoms adsorbed on the HfO2 surface provide electrons for the heterostructure. Some of these electrons transfer to the conduction band near the interface, leading to the formation of a 2DEG in that region. Meanwhile, the remaining electrons stay on the surface, forming a conductive layer with a thickness of approximately 2 unit cells. As the thickness of the HfO2 layer increases, the probability of electrons transferring from the surface to the interface gradually decreases, resulting in a gradual decrease in the electron density at the interface.
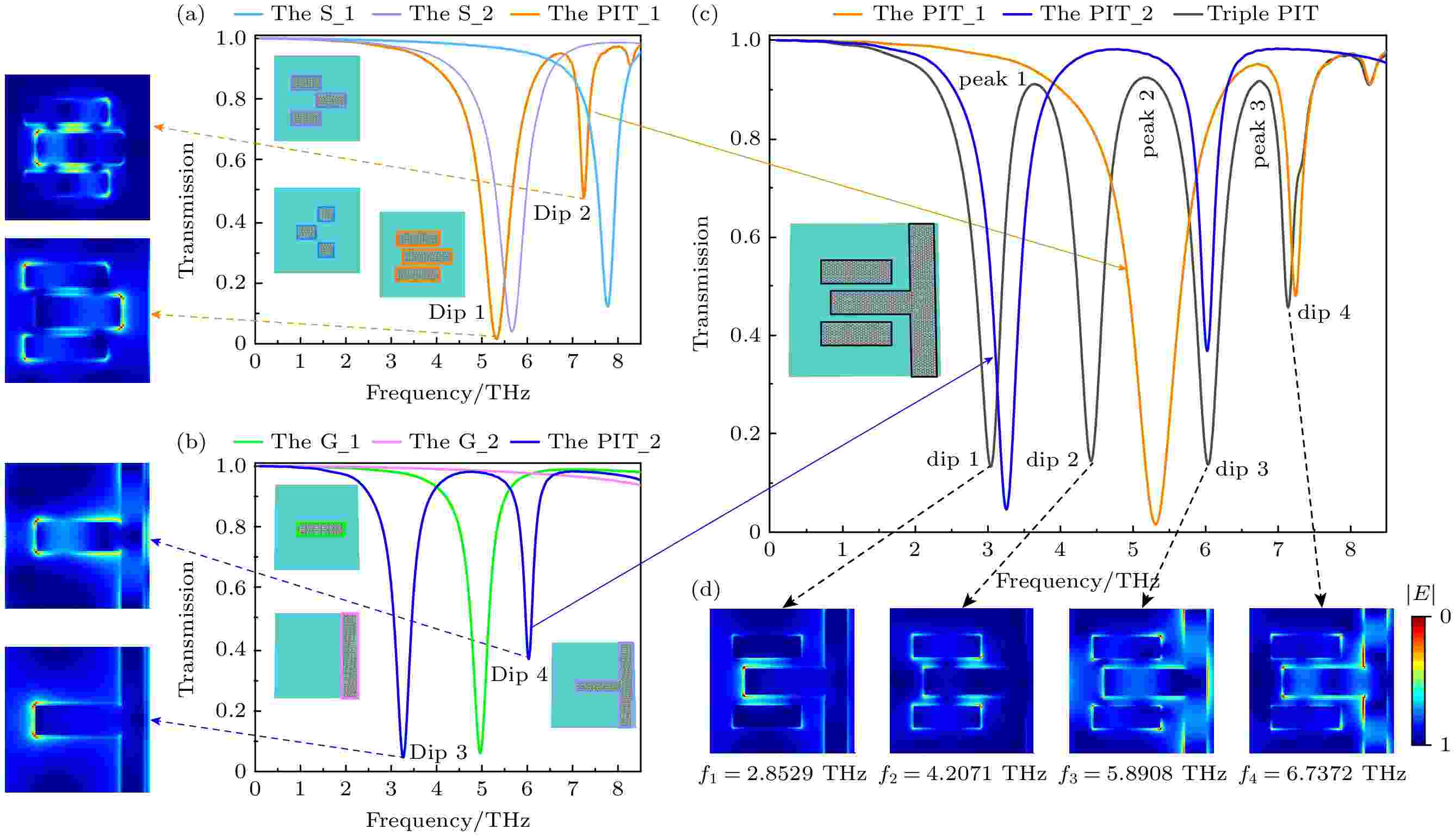
2025, 74 (9): 097801.
doi: 10.7498/aps.74.20250078
Abstract +
Surface plasmons (SPs) are generated by the interaction of conduction electrons on the surface of a metallic medium with photons in light wave, and they have an important phenomenon called plasmon-induced transparency (PIT). The PIT effect is crucial for improving the performance of nano-optical devices by strengthening the interaction between light and matter, thereby enhancing coupling efficiency. As is well known, traditional PIT is mainly achieved through two main ways: either through destructive interference between bright and dark modes, or through weak coupling between two bright modes. Therefore, it is crucial to find a new excitation method to break away from these traditional approaches. In this work, we propose a single-layer graphene metasurface composed of longitudinal graphene bands and three transverse graphene strips, which can excite a tripe-PIT through the synergistic effect between two single-PITs. We then leverage the synergistic effect between these two single-PITs to realize a triple-PIT. This approach breaks away from the traditional method of generating PIT through the coupling of bright and dark modes. The numerical simulation results are also obtained using the finite-difference time-domain, which are highly consistent with the results of the coupled-mode theory, thereby validating the accuracy of the results. In addition, by adjusting the Fermi level and carrier mobility of graphene, the dynamic transition from a five-frequency asynchronous optical switch to a six-frequency asynchronous optical switch is successfully achieved. The six-frequency asynchronous optical switch demonstrates exceptional performance: at frequency points of 3.77 THz and 6.41 THz, the modulation depth and insertion loss reach 99.31% and 0.12 dB, respectively, while at the frequency point of 4.58 THz, the dephasing time and extinction ratio are 3.16 ps and 21.53 dB, respectively. Additionally, when the tuning range is from 2.8 THz to 3.1 THz band, the triple-PIT system exhibits a remarkably high group index of up to 1212. These performance metrics exceed those of most traditional slow-light devices. Based on these results, the structure is expected to provide new theoretical ideas for designing high-performance devices, such as optical switches and slow-light devices.
INTERDISCIPLINARY PHYSICS AND RELATED AREAS OF SCIENCE AND TECHNOLOGY
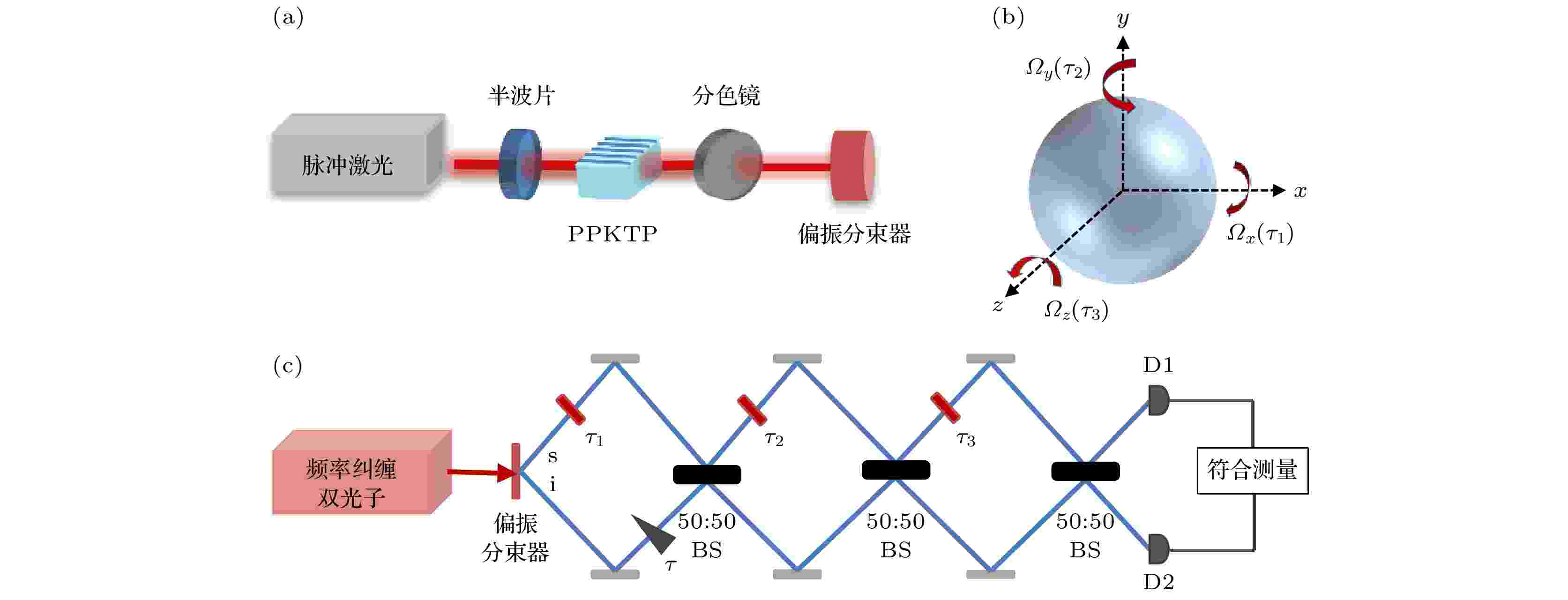
2025, 74 (9): 098501.
doi: 10.7498/aps.74.20250077
Abstract +
The optical gyroscope for measuring the attitude information of spatial carriers, has emerged as a research hotspot in inertial navigation system. Real-time measurement of rotation angular velocity is crucial for obtaining accurate attitude information. However, the measurement accuracy of traditional optical gyroscope is limited by the short noise limit (SNL), which restricts its further applications. Existing research indicates that the quantum technology is needed to address the measurement limitations of traditional optical gyroscopes. A triaxial rotation angular velocity measurement scheme based on frequency entangled biphoton and cascaded Hong-Ou-Mandel (HOM) interference is proposed in this study. By using the Sagnac effect induced by the rotation between signal and idler photons, the triaxial angular velocity is introduced into the corresponding measurement arm of a cascaded HOM interferometer. The cascaded HOM interferogram is obtained using a coincidence measurement device, and the relationship between the symmetric dip position and the three independent time delay difference is analyzed. The characteristic parameters of HOM interferogram, including a half-height full width (FWHM) of 0.3 ps and visibilities of 1, 0.25 and 0.06, respectively, are obtained. According to quantum Fisher information theory, the maximum quantum Fisher information values of the three independent time delay differences ($ {\tau }_{1} $, $ {\tau }_{2} $, $ {\tau }_{3} $) are calculated to be 1, 0.1, and 0.006, respectively. Furthermore, by incorporating measurement uncertainty, it is demonstrated that the accuracy of the time delay measurement can exceed the SNL. Combined with the relationship between time delay and angular velocity, the results show that the angular velocity measurement accuracy exceeds that of classical optical gyroscopes. Therefore, this scheme provides a theoretical foundation for further applying quantum gyroscopes to global navigation sensing and precision measurement systems.
RETRACTION NOTICE
2025, 74 (9): 099901.
doi: 10.7498/aps.74.099901
Abstract +
The article titled “Study on the electronic structure and photocatalytic properties of a novel monolayer TiO2” by Ziqian Xiong et al., published in Acta Physica Sinica (Vol. 69, Issue 16: 166301, DOI: 10.7498/aps.69.20200631 ) in 2020, contains errors in the data processing related to the calculation of elastic constants and mobility. These inaccuracies have led to incorrect results in the paper. In order to ensure the accuracy and reliability of the research findings and to prevent misleading information from impacting the academic community, the authors have decided to request the retraction of this paper. This statement is issued to formally announce this decision.